Data on production and economic impacts at scale for the Viet-Uc commercial shrimp producer partnering with CSIRO
Research to improve aquaculture production systems is often performed outside commercial settings in smaller, experimental-scale units, over shorter time periods and with a focus on a specific aspect of production. This approach is often necessary to allow control of variables and high treatment replication, but also to adhere to research budgets. However, results can sometimes be difficult to translate to commercial conditions because of the added complexity of commercial environments that can influence the outcomes which respond differently to experimental-scale systems.
Since 2010, CSIRO (The Commonwealth Scientific and Industrial Research Organisation) has collaborated with Viet-Uc Seafood Corporation in various areas, including health management, postlarvae production and genetic improvement of whiteleg shrimp (Litopenaeus vannamei), and selective breeding of black tiger prawn (Penaeus monodon) and pangasius. More recently, a collaborative three-year project ending in 2021 delivered a sustainable and profitable super-intensive shrimp farming system by developing new management and technological approaches tailored to the environmental conditions in Vietnam’s Mekong Delta.
Targeted experiments were designed to evaluate the efficiency and effectiveness of different systems [e.g., biofloc technology (BFT) and clear-water technology]. A range of protocols evaluated for the different systems were designed to maintain optimal levels of key water quality parameters, as well as to (i) assess shrimp performance and pond conditions under a range of stocking densities from 150 up to 600 shrimp per square meter; (ii) refine/implement partial harvesting protocols; (iii) develop strategies to minimize pathogenic vibrio concentrations; (iv) evaluate different commercial supplements and diets, including diets based on the commercial microbial biomass product Novacq™; and (v) design strategies to reduce production costs.
Experimental setup
Six experiments were conducted within two commercial greenhouses over the three years of the project. The experiments evaluated protocols across different seasons, with each experiment running for 90 to 100 days of culture. Each greenhouse contained 14 plastic-lined ponds (500 square meters), which allowed for four to six replicate ponds per treatment. Data was collected and analyzed from 132 commercial ponds in total. Experimental designs systematically refined and retested systems, with new protocols developed based on knowledge gained from the preceding experiments.
Collaboration drives innovations in super-intensive indoor shrimp farming
The project compared clear-water (through water exchange) and biofloc technology (BFT) systems in Experiments 1 and 2 and focused exclusively on BFT after Experiment 2 due to consistently better shrimp performances along with better water quality and significantly lower water use. A comprehensive data collection regime was implemented to enable in-depth analysis of system performance and identification of critical constraints on production. Twenty-two different water quality parameters were routinely monitored in each experiment. The research covered other important aspects of farming and production such as assessing shrimp health, pathogen monitoring and biosecurity, investigating precision farming strategies such as new sensor technologies, data management, machine learning and decision support tools and economic modelling of farming profitability. In a previous article, we provided a summary of the project goals and areas of impact. In this second article we present more details related to the production and economic outcomes at scale.
Enhancing production parameters, water and shrimp quality
Consistency in water quality is critical because it provides stable conditions for shrimp growth, efficient feed utilization and a more predictable environment. This latter benefit reduces monitoring and increases the potential for automation, thereby providing costs savings in labor. In our project, the performance of pond replicates was very consistent, demonstrating accurate execution of protocols which allowed reliable comparison between treatments. This enabled our team to identify the main production constraints and develop targeted solutions to systematically improve production. After the third experiment, key water quality parameters were stable and within optimal ranges for L. vannamei for all tested protocols.
CSIRO-Viet Uc, Table 1
Parameter | Initial protocols (2018) | Final protocol (2020) | |||
---|---|---|---|---|---|
Experiment 1 | Experiment 2 | Experiment 6 (BFT only) | |||
BFT | CW | BFT | CW | ||
Yield (ton/ha/cycle) | 27.0 (24-30) | 18.5. (15-22) | 32.3 (32-33) | 24.5 (24-25) | 43.3 (39-46) |
Survival (%) | 81.0 (70-92) | 63.5 (60-67) | 86.6 (86-87) | 66.3 (60-72) | 94.7 (82.7-99.1) |
FCR | 1.4 (1.2-1.5) | 1.9 (1.9-1.9) | 1.3 (1.2-1.3) | 1.4 (1.3-1.4) | 1.3 (1.2-1.5) |
TAN (mg/L)1 | 0.2 (0.9) | 2.3 (7.3) | 0.11 (0.5) | 0.54 (2.41) | 0.13 (0.30) |
Nitrite (mg/L)1 | 12.3 (56.6) | 12.7 (44.7) | 2.66 (12.85) | 13.2 (45.3) | 1.28 (5.41) |
Vibrio (green vibrio: total bacteria ratio)2 | 1.9 (1.3-2.6) | 7.3 (6.8-7.9) | 6.0 (5.9-6.1) | 12.0 (9.0-15.0) | 0.1 (0-0.1) |
Water use (L/kg of shrimp) | 467 (416-519) | 6,158 (5,164-7,153) | 378 (376-380) | 4,756 (3,343-6,169) | 674 (624-812) |
Data presented as average (all ponds) and maximum and minimum (by treatment) 1 Average (all ponds) and maximum value (peak) observed in a particular treatment 2 Project target < 5%
Throughout the project, increasing and consistent shrimp yields were achieved in BFT systems. During the last experiment, consistent harvest yields of 43 t/ha/cycle were achieved under a range of different biofloc protocols (Fig. 3). From experiment 2 onward, survival increased and averaged over 80 percent for all BFT protocols, with some treatments averaging over 90 percent across all replicate ponds in experiments 5 and 6 (Fig. 3).
A feed conversion ratio (FCR) value of less than 1.3 was frequently achieved. Experiment 5 produced FCRs below 1.25 in some treatments and below 1.20 at a density of 150 shrimp per square meter (Fig. 4). This is a key outcome for the future sustainability of commercial shrimp production, both in terms of cost efficiency and to minimize waste. The biofloc protocol enabled significantly less water use than the clear water protocol. In experiment 6, water use averaged 674 L/kg of shrimp produced. The best clear-water protocols (experiments 1 and 2) ranged from 3,343 to 5,164 L/kg of shrimp produced (Fig. 4).
The greenhouse infrastructure and management practices were refined to provide optimal water temperatures (27 to 30 degrees-C) for shrimp growth, especially during winter crops. The biofloc protocol combined with the indoor environment increased biosecurity and provided stable water quality conditions with minimal daily fluctuations in temperature, dissolved oxygen (> 5 mg/L) and pH (~7.8). The salinity varied from approximately 10 to 35 ppt depending on the season. Alkalinity was maintained at levels higher than 150 mg/L. Turbidity, settling solids and total suspended solids were kept below 50 Formazin Nephelometric Unit (FNU), 5 mL/L and 250 mg/L, respectively, in the last two experiments. Continual improvements in the BFT protocol using a chemoautotrophic-based strategy resulted in greater control over toxic nitrogen compounds (total ammonia nitrogen and nitrite (Fig. 5).
The project experienced challenges in the form of pathogenic vibrio in the water and early-stage health issues for shrimp in experiments 2 and 3, respectively. These challenges were overcome through a combination of approaches that were implemented over the course of the project and were useful in exploring solutions commonly faced by shrimp producers. Approaches included developing specific protocols for pathogenic vibrio control, adjusting pond management procedures such as siphoning and water exchanges, using water supplements (e.g., probiotics and prebiotics), sanitation products and applications of minerals, and improving intake water settling, filtration and sanitation protocols.
The proportion of total vibrio and green colony vibrio (potentially pathogenic) was drastically reduced from 15 to 30 percent (peak levels observed in experiments 3 and 4, respectively) to less than 0.5 percent of total bacteria in the latter experiments, resulting in higher survival and superior shrimp quality (Fig. 6). The project target was to maintain levels of green colony vibrio to total bacteria below 5 percent, likely indicating a favorable bacteria balance within the production units.
Economics
Super-intensive indoor systems can support enhanced biosecurity and disease mitigation, leading to more consistent production performance compared to traditional outdoor operations. However, the establishment and ongoing production costs of indoor systems are higher.
To address this challenge, the project conducted economic analyses to identify the various influences on profitability. Subsequent economic models were used to run simulations and identify system and management modifications to optimize economics returns. The resulting protocol adjustments were tested and resulted in a 13 percent increase in the Net Profit Margin (NPM) across the final three experiments when compared to the baseline protocol.
Impact at scale
Our project highlights the benefits of performing production system research directly at the farm and taking a whole-of-system approach. This approach allows us to evaluate the direct influence that specific treatments are having, but also the indirect influences that occur in a larger environment over a longer time period. As we demonstrated, this research model can deliver substantial improvements in production and economics for a commercial company.
The key to success is a trusting relationship between the scientists, the company and its staff, and a willingness of the company to invest a significant amount of time and resources into R&D. This initiative is critical to the mission of CSIRO to develop, evaluate and deploy new technologies, generating impact at scale. The CSIRO team wishes to acknowledge and thank Viet-Uc for their continued support, contribution and dedication to the project.
Now that you've reached the end of the article ...
… please consider supporting GSA’s mission to advance responsible seafood practices through education, advocacy and third-party assurances. The Advocate aims to document the evolution of responsible seafood practices and share the expansive knowledge of our vast network of contributors.
By becoming a Global Seafood Alliance member, you’re ensuring that all of the pre-competitive work we do through member benefits, resources and events can continue. Individual membership costs just $50 a year.
Not a GSA member? Join us.
Authors
-
Mauricio G.C. Emerenciano, Ph.D.
Corresponding author
Commonwealth Scientific and Industrial Research Organisation (CSIRO)
Bribie Island Research Centre
144 North Street, Woorim, Qld. Australia[117,97,46,111,114,105,115,99,64,111,110,97,105,99,110,101,114,101,109,101,46,111,105,99,105,114,117,97,109]
-
Stuart Arnold
Commonwealth Scientific and Industrial Research Organisation (CSIRO)
Bribie Island Research Centre
144 North Street, Woorim, Qld. Australia[117,97,46,111,114,105,115,99,64,100,108,111,110,114,97,46,116,114,97,117,116,115]
-
Tim Perrin
Commonwealth Scientific and Industrial Research Organisation (CSIRO)
Bribie Island Research Centre
144 North Street, Woorim, Qld. Australia -
Bryce Little
Commonwealth Scientific and Industrial Research Organisation (CSIRO)
Queensland Bioscience Precinct
306 Carmody Road, St. Lucia, Qld. Australia -
Jeff A. Cowley, Ph.D.
Commonwealth Scientific and Industrial Research Organisation (CSIRO)
Queensland Bioscience Precinct
306 Carmody Road, St. Lucia, Qld. Australia -
Ashfaqur Rahman, Ph.D.
Commonwealth Scientific and Industrial Research Organisation (CSIRO)
Sandy Bay Site
15 College Road, Sandy Bay, Tasmania, Australia
Tagged With
Related Posts
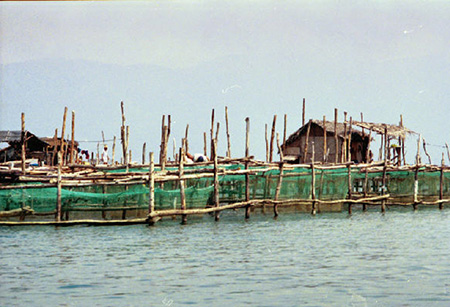
Aquafeeds
CSIRO studies dry feeds for juvenile spiny lobsters
The Commonwealth Scientific and Industrial Research Organisation has researched pelleted dry feeds are palatable to juvenile tropical spiny lobsters.
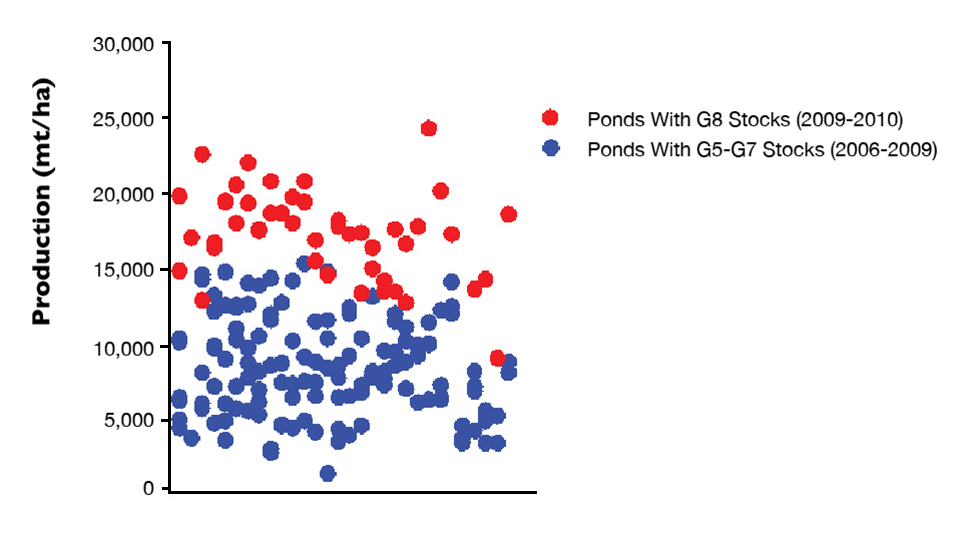
Health & Welfare
Black tiger breeding program yields record shrimp harvests in Australia
Traditionally, Australian farmers relied on wild broodstock to source black tiger shrimp larvae, but substantial progress has been made in the domestication and selective breeding of Australian P. monodon.
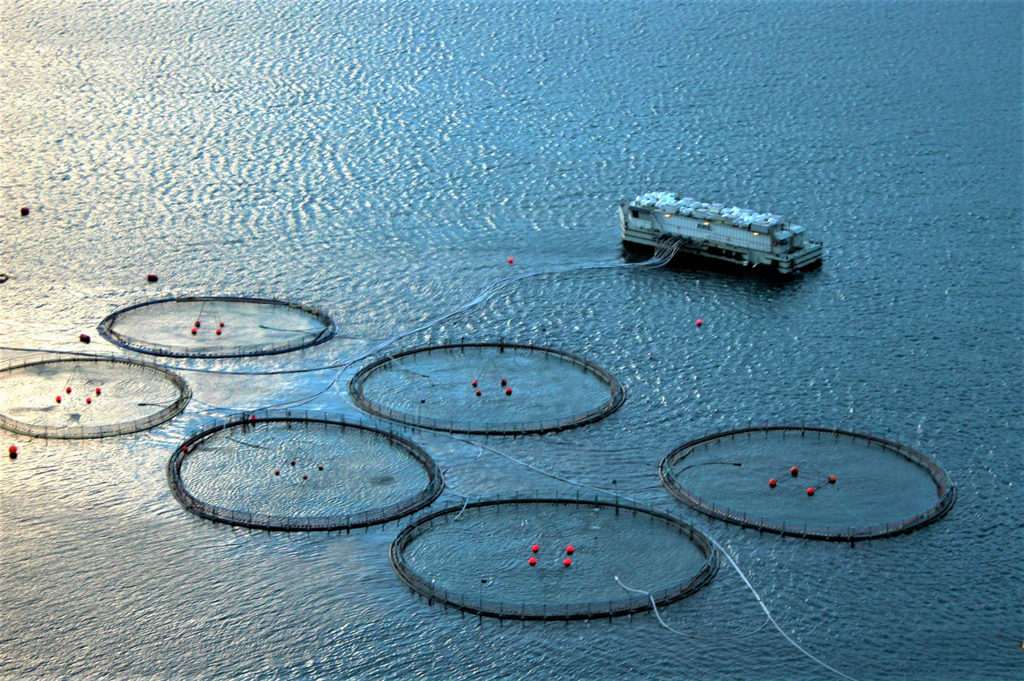
Responsibility
Precision fish farming: A new framework to improve aquaculture, Part 2
Proof-of-concept studies for precision fish farming would be easier in laboratories, but demonstrating effects in full-scale farming conditions is critical.
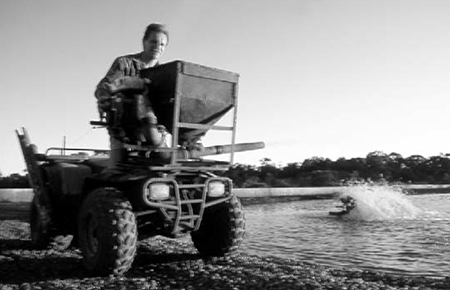
Aquafeeds
Shrimp feed management in Australia
A shrimp feed management survey indicated that Australian farmers placed a high importance on better estimations of feed demand in improving FCR.