Shrimp obtain additional nutrients from biofloc, leading to improved growth

Super-intensive production of shrimp is gaining increased attention worldwide as a potential means to improve aquaculture production via its application as a transitional nursery system between the hatchery and growout ponds. However, a significant increase in stocking density in the nursery phase can decrease the growth and survival of the shrimp because of the decreased space and availability of natural food, cannibalism, degradation of water quality and accumulation of organic matter on the bottoms of tanks.
This situation can require approaches such as culture systems with biofloc technology. These systems include predominantly aerobic and heterotrophic bacteria, protozoa, metazoans, microalgae, exoskeletons, feces and the remains of dead organisms.
The ability of shrimp to obtain additional nutrients from biofloc has been suggested as one of the reasons for the improved growth of Pacific white shrimp (Litopenaeus vannamei) reared in biofloc systems.
Study setup
To evaluate the effects of stress caused by population density on L. vannamei postlarvae under high-density biofloc culture, the authors performed an experiment in the indoor tank facilities of the Marine Aquaculture Station of the Federal University of Rio Grande, Rio Grande do Sul, Brazil. The biological material used was acquired from the Aquatec Ltda. laboratory in Canguaretama, Rio Grande do Norte, Brazil.
After the L. vannamei nauplii arrived, they were kept in the sector of the hatchery for marine shrimp until reaching the P.L.25 postlarval stage. With mean initial weights of 0.009 ± 0.002 grams, the shrimp postlarvae were subsequently transferred to the experimental tanks, where the experimental design was randomized with treatments reflecting four stocking densities: 1,500; 3,000; 6,000 and 9,000 shrimp/cubic meter. Three replicates were randomly assigned to each stocking density.
The shrimp were fed twice daily with commercial 40 percent protein feed. Initially, the feeding rate was established according to other authors. Later, the feed was adjusted daily according to the consumption of the shrimp. The study lasted 42 days.
The study system included 12, 0.15-cubic-meter circular microcosm tanks that received intense aeration with blowers. Each experimental tank received water pumped from a 4-cubic-meter macrocosm tank. This water returned via gravity to a drain directed to the macrocosm tank. The water was completely recirculated from the macrocosm matrix tank to the microcosms about 20 times each day.
The tanks were filled with water pumped from a beach and filtered through a sand filter and cartridge filter. Before starting the study, the matrix tank of the system was inoculated with water containing bioflocs from a rearing system for shrimp used in another experiment. It was stocked with 34 shrimp/cubic meter to enhance the formation and maintenance of bioflocs.
There was no renewal of water during the study, only replacement of what was lost due to evaporation by adding dechlorinated freshwater. During the experiment, the use of sugar cane molasses as a source of organic carbon was not necessary because the levels of total ammonia did not reach 1 mg/L.
The chosen photoperiod for the experimental room was 12 hours of light and 12 hours of darkness, maintained by artificial lighting. The water temperature was maintained with two heaters immersed in the matrix tank. During the study development, physicochemical parameters were monitored in both the matrix and experimental tanks.
During the study, 50 shrimp from each experimental tank were weighed individually using a digital balance. The shrimp were returned to their original tanks after weighing. At the study end, the surviving shrimp were weighed and counted to evaluate their growth and survival.
Results
Fig. 1 shows the fluctuations of the water quality in the matrix tank and biofloc culture system. The physicochemical parameters monitored throughout the study did not present significant differences among the treatments and their respective matrix tanks. During the study, the total ammonia concentration did not exceed 1.5 mg/L. High nitrate (85.0 ± 24.9 mg/L) and total suspended solids (711.0 ± 58.7 mg/L) concentrations were seen in the biofloc culture system.
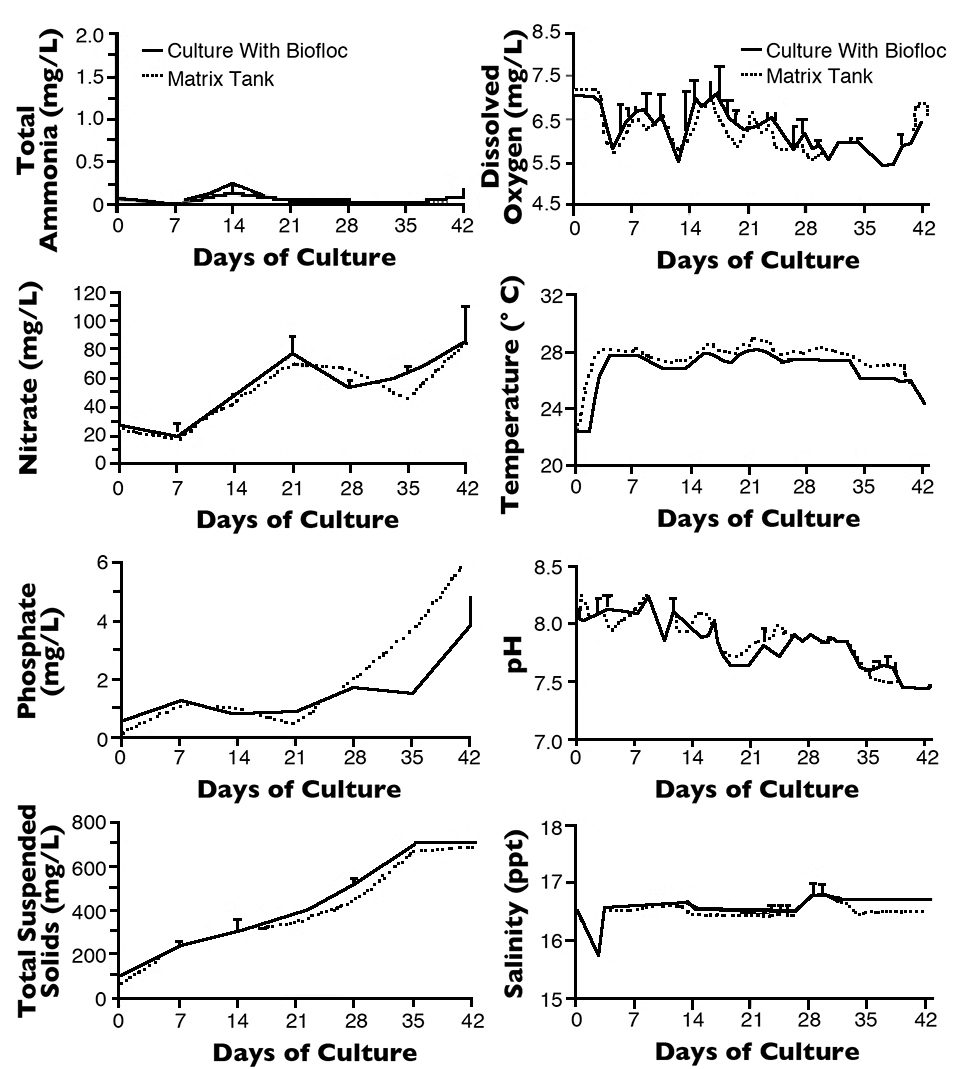
At the end of the experiment, the shrimp reared in the biofloc system had significant differences in mean final weight among the treatments (Fig. 2). But there was no significant difference between results for the treatments at 6,000 and 9,000 shrimp per cubic meter. High survival was observed and there was no significant difference among the stocking densities (Fig. 3).
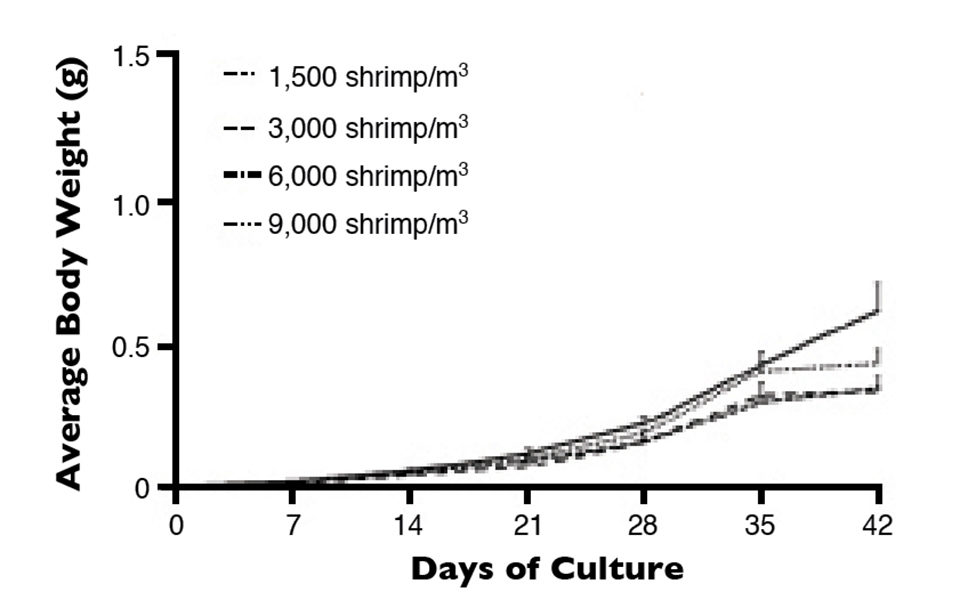
The produced biomass values were significantly different among the treatments. At the lower densities of 1,500 and 3,000 shrimp/cubic meter, no significant difference occurred. The biomass values did increase with an increase in stocking density. The productivity range was 0.9-2.5 kg per cubic meter.

(Editor’s Note: This article was originally published in the July/August 2015 print edition of the Global Aquaculture Advocate.)
Authors
-
Héctor M. Esparza-Leal, Ph.D.
Departamento de Acuacultura
Instituto Politécnico Nacional
CIIDIR Unidad Sinaloa
Boulevard Juan de Dios Bátiz Paredes #250
Guasave, Sinaloa 81101 Mexico[120,109,46,110,112,105,64,97,122,114,97,112,115,101,104]
-
Alessandro Pereira Cardozo, Ph.D.
Universidade Federal do Rio Grande
Instituto de Oceanografia
Laboratório de Carcinocultura
Rio Grande, Estado do Rio Grande do Sul, Brasil -
Wilson Wasielesky, Jr., Ph.D.
Universidade Federal do Rio Grande
Instituto de Oceanografia
Laboratório de Carcinocultura
Rio Grande, Estado do Rio Grande do Sul, Brasil
Tagged With
Related Posts
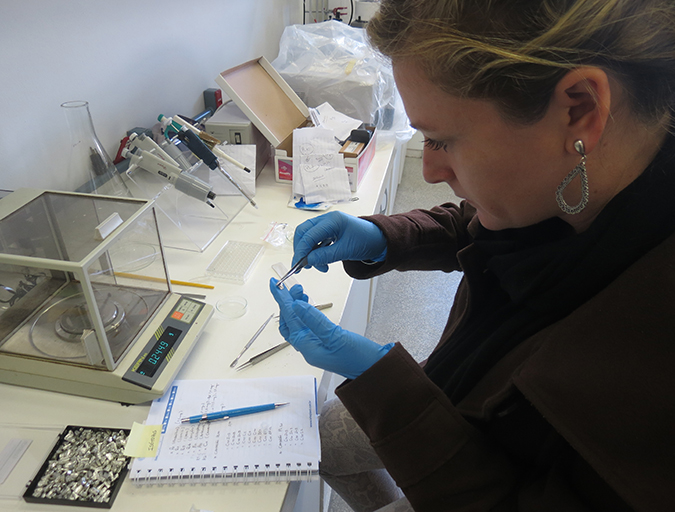
Aquafeeds
Biofloc consumption by Pacific white shrimp postlarvae
The stable isotopes technique with δ13C and δ15N can be used to determine the relevance of different food sources to shrimp feeding during the pre-nursery phase of Litopenaeus vannamei culture. During this trial, different types of commercial feed, microalgae, Artemia sp. nauplii and bioflocs were used as food sources.
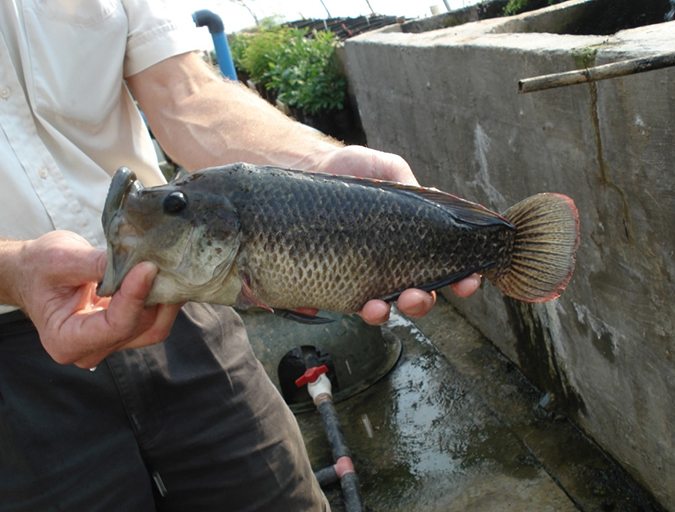
Aquafeeds
Biofloc systems viable for tilapia production
Well-designed and managed biofloc technology systems are a viable alternative for production of various species like tilapia, to increase feed efficiency by lowering aquafeed protein requirements and to help reduce or eliminate effluent discharges.
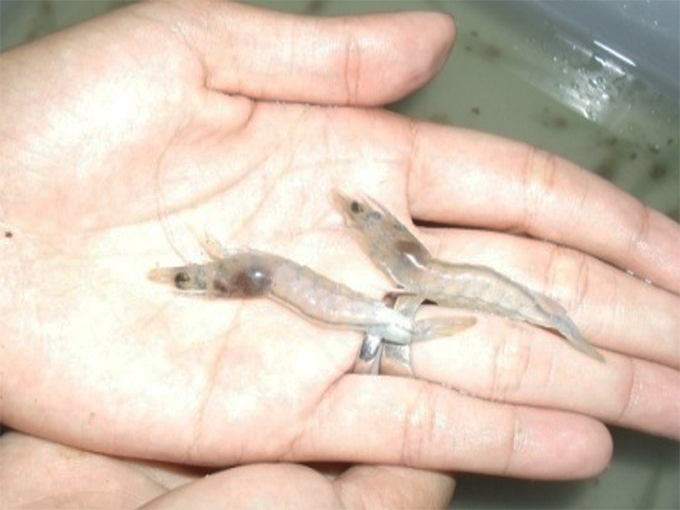
Aquafeeds
Rotifers and diatoms aid shrimp biofloc nurseries
Larval shrimp performance (feed conversion and final weight) in a biofloc nursery system improved with both added diatoms (Navicula sp.) and rotifers (Brachionus plicatilis).
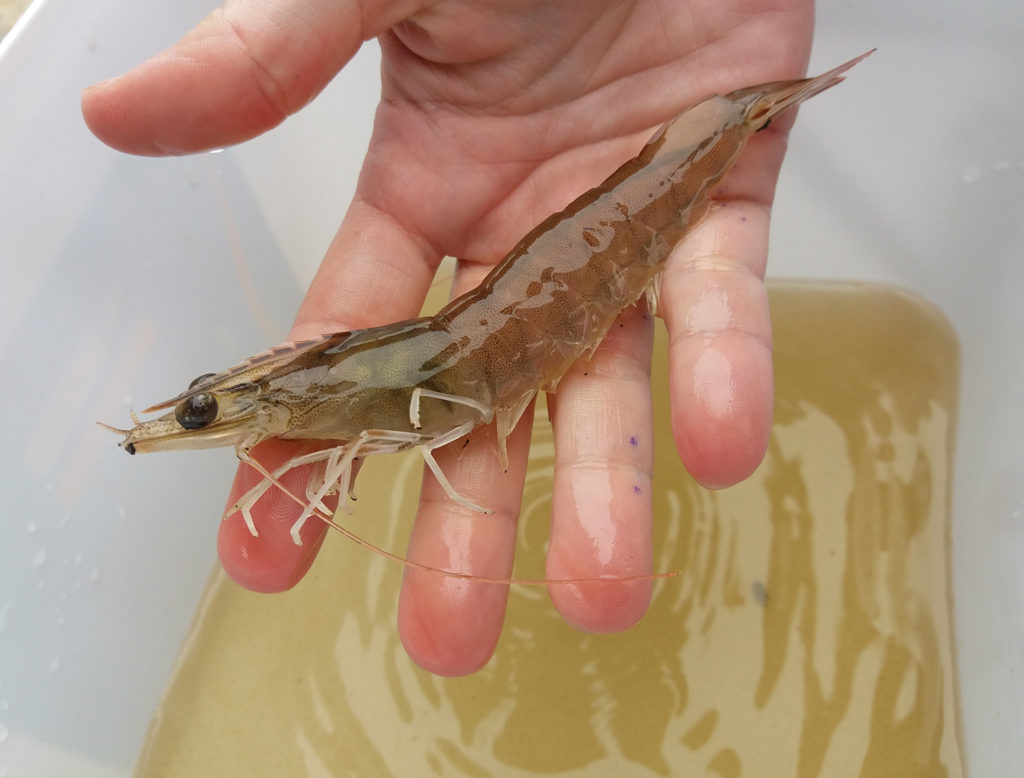
Aquafeeds
What is the best carbon-nitrogen ratio for biofloc systems?
A recent study at the Federal University of Rio Grande in Brazil showed that it is possible to reduce the C:N ratio in biofloc cultures, to decrease the water volume used and the total suspended solids produced, with resulting savings.