Use conservative feeding, aeration and liming to mitigate impacts
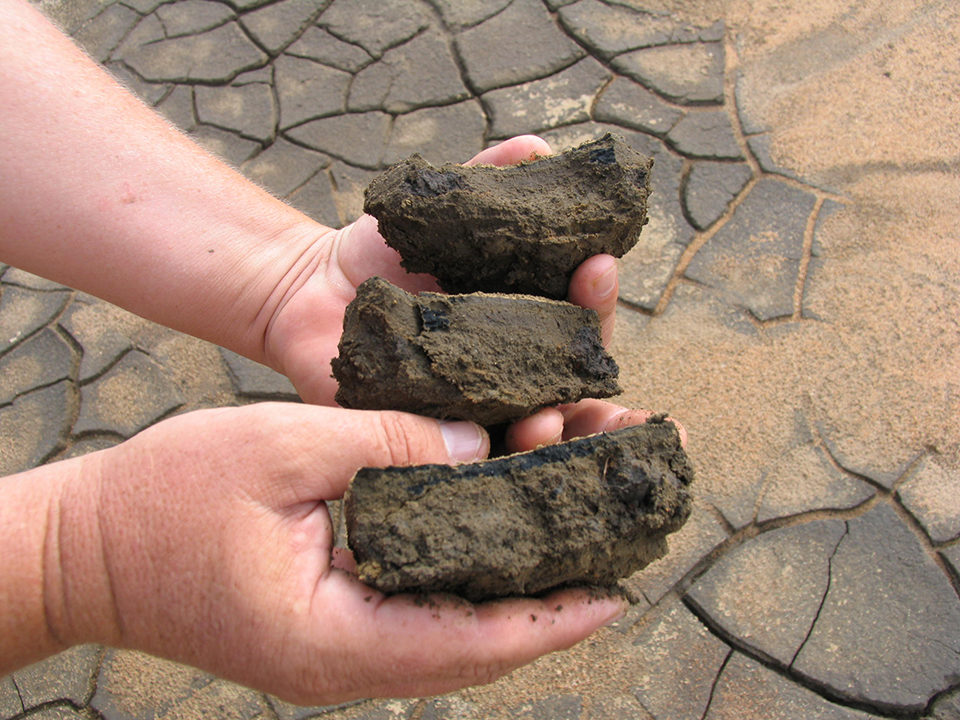
Sulfur is an essential element for plants, animals and bacteria. It is present in natural waters and water of aquaculture systems, mainly as the sulfate ion. In humid regions, sulfate concentrations in water usually are 5 to 50 mg/L, but in arid regions, concentrations often exceed 100 mg/L. Seawater contains 2,700 mg/L of sulfate, on average. Although sulfate is rarely applied to aquaculture systems specifically for increasing ambient concentrations, it is present in feed and a few water quality amendments.
Issues in aquaculture
The main sulfur-related issue in aquaculture is the occasional presence of toxic concentrations of hydrogen sulfide. Sulfide can occur in water because it is a metabolite of Desulfovibrio and certain other bacteria found in anaerobic zones – usually in sediment. These bacteria use oxygen from sulfate as an alternative to molecular oxygen in respiration.
There are three forms of sulfide (H2S, HS– and S2-), and they exist in a pH- and temperature-dependent equilibrium. The effect of pH on the distribution of the three forms at 25 degrees-C is shown in Fig. 1. As pH increases, the proportion of hydrogen sulfide declines, and that of HS– rises until the two forms have roughly equal proportions at pH 7. At greater pH, HS- is the dominant form, and there is no S2- until the pH is above 11.

Hydrogen sulfide is toxic to aquatic animals because it interferes with reoxidation of cytochrome a3 in respiration. This effect is caused almost entirely by H2S, while HS- is essentially non-toxic. Even if it is toxic, S2- is not an issue, because it does not occur at pH values found in aquaculture systems.
Hydrogen sulfide concentration
The concentration of hydrogen sulfide must be estimated from total sulfide concentration, because methods for determining sulfide in water typically measure the total concentration of the three sulfide forms.
The proportions of H2S at different pH values and temperatures provided in Table 1 can be used for estimating hydrogen sulfide concentration. To illustrate, suppose the pH is 7.5 at 26 degrees-C in freshwater with a sulfide concentration of 0.5 mg/L. The factor for these conditions is 0.238. Multiplying the factor by the sulfide concentration of 0.5 mg/L gives an H2S concentration of 0.119 mg/L. In seawater of the same temperature and pH, the concentration would be less by a factor of 0.9.
Boyd, Factors for estimating hydrogen sulfide concentration, Table 1
Temperature (° C) pH | Temperature (° C) 16 | Temperature (° C) 18 | Temperature (° C) 20 | Temperature (° C) 22 | Temperature (° C) 24 | Temperature (° C) 26 | Temperature (° C) 28 | Temperature (° C) 30 | Temperature (° C) 32 |
---|---|---|---|---|---|---|---|---|---|
5.0 | 0.993 | 0.992 | 0.992 | 0.991 | 0.991 | 0.990 | 0.989 | 0.989 | 0.989 |
5.5 | 0.977 | 0.976 | 0.974 | 0.973 | 0.971 | 0.969 | 0.967 | 0.965 | 0.963 |
6.0 | 0.932 | 0.928 | 0.923 | 0.920 | 0.914 | 0.908 | 0.903 | 0.897 | 0.891 |
6.5 | 0.812 | 0.802 | 0.792 | 0.781 | 0.770 | 0.758 | 0.746 | 0.734 | 0.721 |
7.0 | 0.577 | 0.562 | 0.546 | 0.530 | 0.514 | 0.497 | 0.482 | 0.466 | 0.450 |
7.5 | 0.301 | 0.289 | 0.275 | 0.263 | 0.250 | 0.238 | 0.227 | 0.216 | 0.206 |
8.0 | 0.120 | 0.114 | 0.107 | 0.101 | 0.096 | 0.090 | 0.085 | 0.080 | 0.076 |
8.5 | 0.041 | 0.039 | 0.037 | 0.034 | 0.032 | 0.030 | 0.029 | 0.027 | 0.025 |
9.0 | 0.013 | 0.013 | 0.012 | 0.011 | 0.010 | 0.010 | 0.009 | 0.009 | 0.008 |
Sulfide in sediment
Hydrogen sulfide formation in sediment is mainly the result of sulfate reduction by microorganisms. Sulfate reduction occurs at a lower oxidation-reduction (redox) potential than is necessary for the reduction of iron and manganese by microorganisms. Thus, ferrous iron and manganous manganese usually are present in zones where hydrogen sulfide is produced.
Iron, manganese and other metals quickly react with hydrogen sulfide to form highly insoluble metallic sulfides that precipitate. This process usually lessens the hydrogen sulfide concentration in sediment, but over 100 mg/L of hydrogen sulfide has been reported in some sediments.
Hydrogen sulfide in sediment can enter overlying water by diffusion. It also can be mixed into the water column by biological activity and sediment disturbances by seine hauls and strong water currents caused by wind or mechanical aeration. If the rate at which hydrogen sulfide enters the water exceeds the rate of its oxidation, there will be a detectable concentration of this potential toxin in the water column – especially in the layer a few centimeters above the sediment-water interface.
Toxicity
The 96-hour lethal concentration 50 (LC50) values for hydrogen sulfide to freshwater fish species range 20-50 μg/L, and much lower concentrations stress fish and make them more susceptible to disease. A measure of toxicity, LC50 reflects the concentration of a compound in water that killed 50 percent of the test animals in a specified period of time, e.g., 96-hour LC50.
Ideally, freshwater fish should not be exposed to more than 2 μg/L of hydrogen sulfide for long periods. Shrimp and other marine species tend to be more tolerant of hydrogen sulfide than freshwater species are.
Values for 96-hour LC50s of hydrogen sulfide to marine species range 50-500 μg/L. Nevertheless, hydrogen sulfide concentration probably should not exceed 5 μg/L in aquaculture ponds with brackish water of full-strength seawater. As with freshwater fish, elevated concentrations of hydrogen sulfide increase the susceptibility of marine organisms to disease – especially Vibriosis in the case of shrimp.
Studies in laboratory soil-water systems conducted at Texas A&M University suggested that high sulfide concentrations in sediment pore water did not affect shrimp, provided the soil-water interface remained aerobic, and the dissolved-oxygen concentrations in the water column were at 70 percent saturation or greater. Studies also have shown that the risk of hydrogen sulfide toxicity increases with lower sediment and water pH.
Measurement
Total sulfide concentration measurement is a complex task by standard laboratory methods, but aquaculturists can use hydrogen sulfide kits for easier total sulfide analyses. The kits provide relatively reliable data.
Of course, estimation of hydrogen sulfide concentration from total sulfide concentration requires data on water temperature and pH (Table 1).The presence of hydrogen sulfide often can be detected by its extremely strong, rotten egg odor. Measurable hydrogen sulfide in water usually means a low dissolved-oxygen concentration in the water or at the sediment-water interface, and aeration should be increased.
Management
As mentioned above, aerator-induced water currents can disturb sediment, favoring the mixing of hydrogen sulfide into the water, but the positive benefits of oxygenation by aeration far outweigh this effect. Nevertheless, aerators should be installed in a manner that minimizes the disturbance of sediment.
The main practices for lessening the risk of hydrogen sulfide toxicity are conservative feeding to avoid wasted feed on pond bottoms, plenty of aeration to prevent low dissolved-oxygen levels and provide a flow of oxygenated water across the soil-water interface, and liming to prevent acidic sediment and water. Between crops, pond bottoms should be thoroughly dried. Sediment should be removed from ponds where it is too deep to dry thoroughly, and acidic pond bottoms should be limed.
Some products are sometimes applied to ponds because they can potentially alleviate hydrogen sulfide problems. These include potassium permanganate application to water at a concentration six to eight times the hydrogen sulfide concentration – permanganate can oxidize sulfide. Iron compounds such as ferrous oxide have been applied to sediment at rates of 1 kg/m2 or more to encourage precipitation of hydrogen sulfide in sediment pore water as iron sulfide. Sodium nitrate added to the water column can help maintain oxygenated conditions at the soil-water interface and lessen the opportunity of hydrogen sulfide diffusion into the water.
Probiotics often are added to ponds with the belief that they lessen the risk of hydrogen sulfide toxicity. Sulfur-oxidizing bacteria already are present in ponds, and it is doubtful that probiotic treatments are effective for removing hydrogen sulfide. Zeolite is sometimes claimed to absorb hydrogen sulfide, but the treatment rate necessary for this to be effective would be far too great to be affordable.
(Editor’s Note: This article was originally published in the March/April 2014 print edition of the Global Aquaculture Advocate.)
Author
-
Claude E. Boyd, Ph.D.
Department of Fisheries
and Allied Aquacultures
Auburn University
Auburn, Alabama 36849 USA[117,100,101,46,110,114,117,98,117,97,64,49,101,99,100,121,111,98]
Tagged With
Related Posts
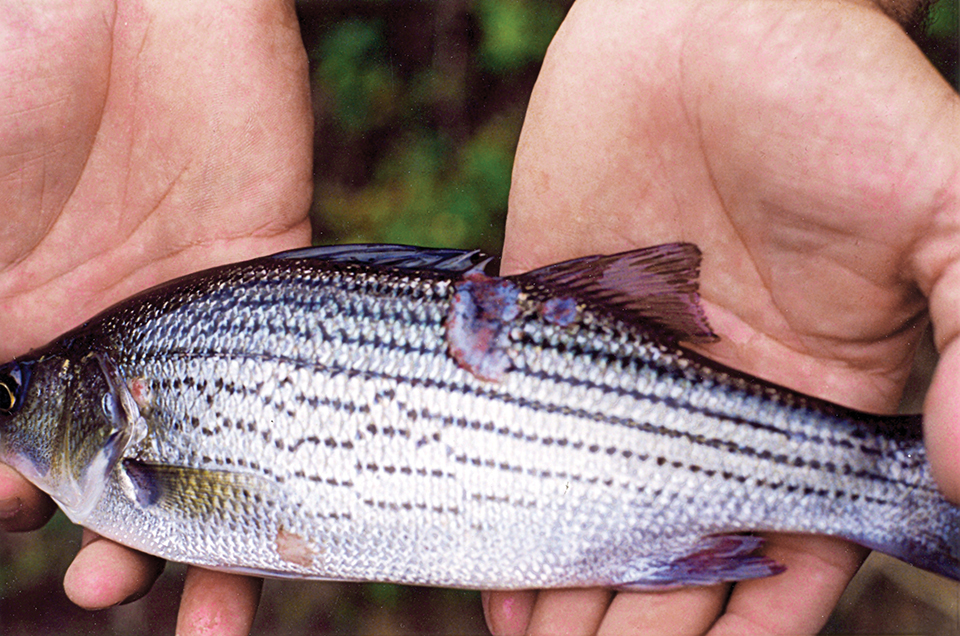
Health & Welfare
Antibiotic-resistant bacteria, part 1
No antimicrobial agent has been developed specifically for aquaculture applications. However, some antibiotic products used to treat humans or land-based animals have been approved for use at aquaculture facilities.
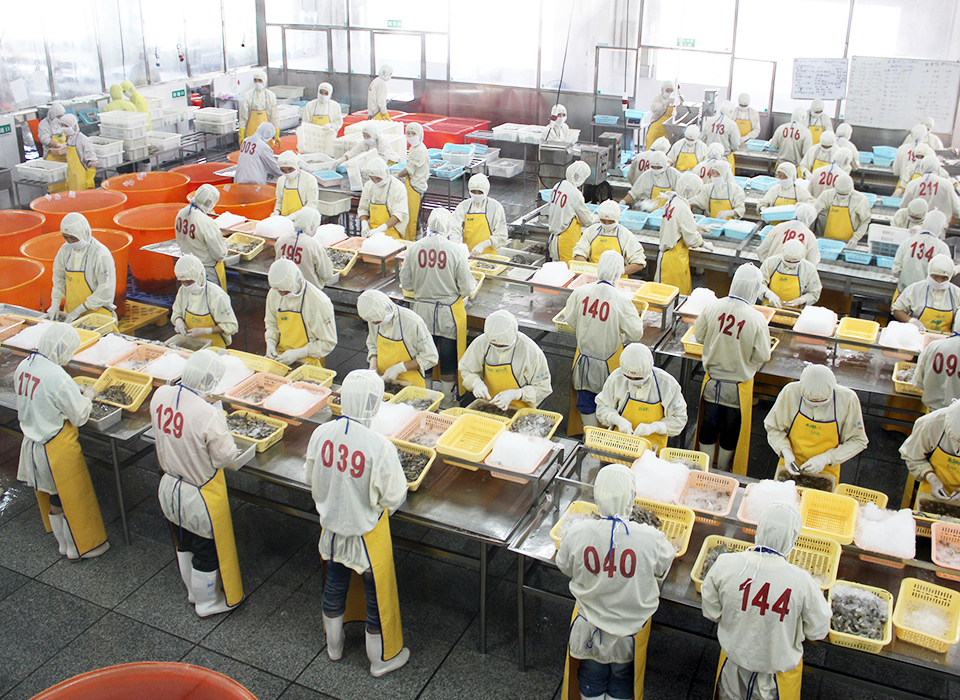
Health & Welfare
Antibiotic-resistant bacteria, part 2
The development of antimicrobial resistance genes in human pathogens as a consequence of exposure to antibiotics in aquaculture is widely documented. Reports implicate foodborne antibacterial-resistant pathogenic bacteria in human disease.
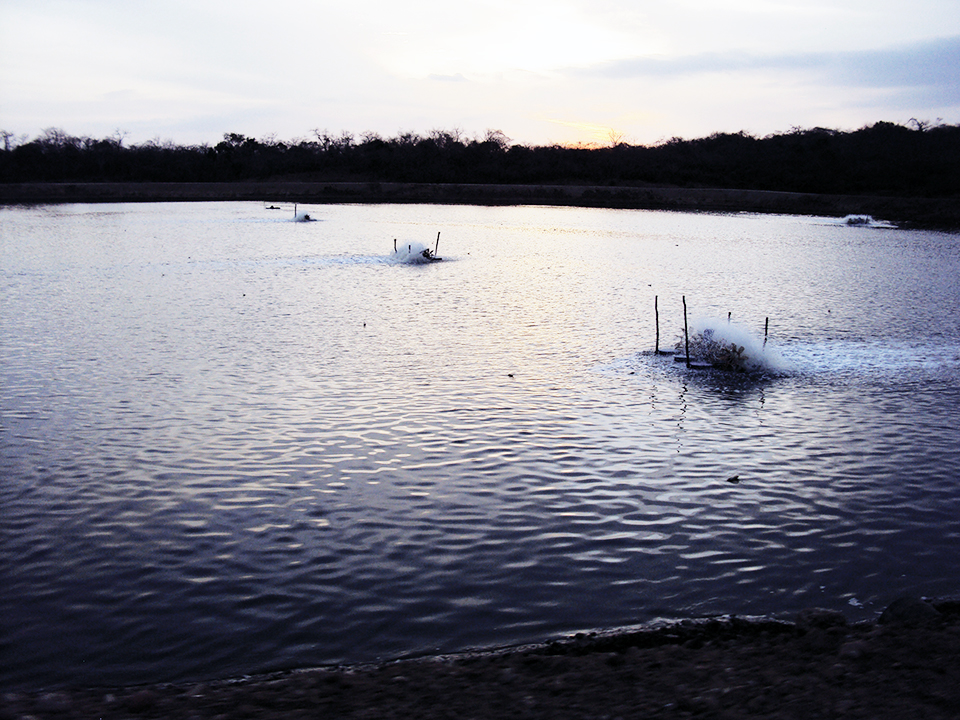
Health & Welfare
Beneficial microbes and pathogen control
A range of alternative products is available to improve animal health and water quality, and control pathogen loads. Beneficial microbes produce antimicrobial compounds and suppress pathogen proliferation.
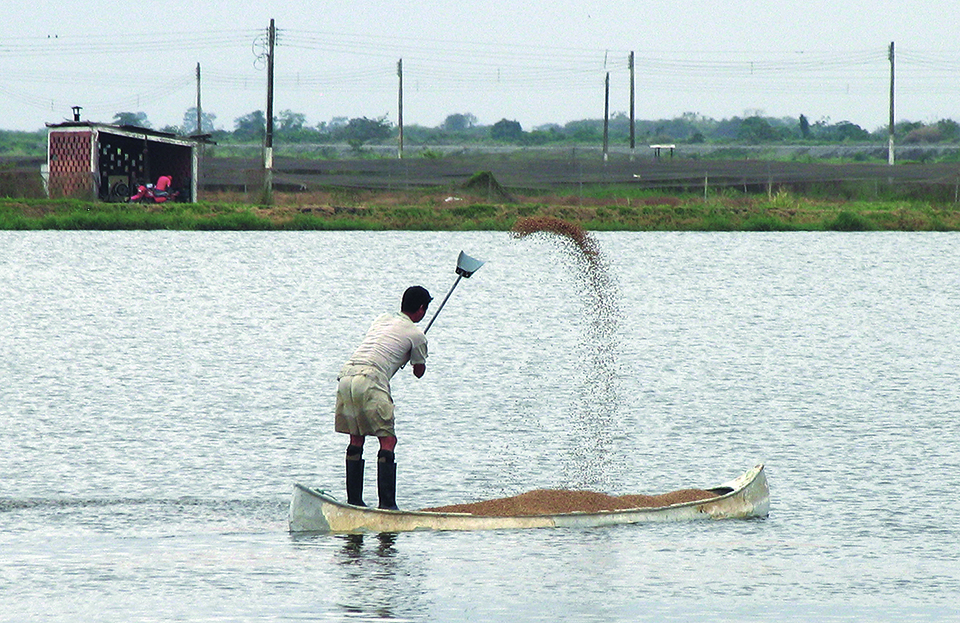
Aquafeeds
Aquaculture feed composition helps define potential for water pollution
A study found that feed for salmon and trout had higher organic carbon concentrations than did catfish, shrimp and tilapia feeds. Nitrogen and phosphorus concentrations were similar among salmon, trout and shrimp feeds, and higher than those in catfish and tilapia feeds.