Why are inexpensive artificial feeds not available for the complete replacement of live feeds?
Hatchery production of the larval stages of marine fish, crustaceans, as well as all the life stages of bivalve mollusks, usually depends on live feeds such as phytoplankton, rotifers, and artemia. Live feeds are expensive to produce and are not always of consistent and dependable quality. Why are inexpensive artificial feeds not available for the complete replacement of live feeds?
The main problems in the development of inexpensive, artificial feeds for suspension feeders have been related to diet delivery rather than diet composition. Small particles (less than 1 mm in diameter) have large surfacearea to volume ratios. Losses of amino acids, water-soluble vitamins and other low molecular weight, water-soluble nutrients are rapid due to high diffusion rates and short diffusion paths through the particle binding material or capsule wall. Researchers have shown, for example, that more than 80 percent of amino acids are lost from alginate- bound microparticles after suspension in water for two minutes.
There have been many attempts to reduce losses of dietary ingredients from microparticles. The most common approach is to bind dietary ingredients in a gel matrix of alginate, carrageenan, zein, or gelatin to produce “microbound” particles (Fig. 1). These dietary particle types are commercially available in powder form. A recent innovation is the commercial production of microbound particles in a slurry or “liquid diet” that can be easily dispersed in the culture medium. Additional ingredients can be added to the liquid diet, such as probiotic bacteria, preservatives and lipid emulsions.
Microencapsulated diets for marine suspension feeders were developed over 20 years ago. The advantage of this approach is that dietary ingredients are surrounded within a capsule wall that may reduce leakage rates of water-soluble nutrients. Commercial production of encapsulated diets is often expensive because the manufacture process is more complicated than for production of microbound particles. The walls of most capsule types also are permeable to low-molecular weight, watersoluble nutrients.
Retention of water-soluble vitamins and amino acids by dietary microparticles can be improved by encapsulating these ingredients in lipid (Fig. 2). Lipid-walled microcapsules consist of a lipid wall surrounding an aqueous core. Water-soluble nutrients can be dissolved in the aqueous core and their leakage rates reduced by the surrounding lipid microcapsule walls. Penaeid shrimp larvae are able to crush capsule walls made of solid tripalmitin with their formidable spiny mouth parts and digestive system. In contrast, marine fish larvae and bivalves require softer-walled lipid capsules in order to break down the walls to release dietary contents. Capsule walls can be softened by adding marine fish oils to tripalmitin, resulting in capsules that supply essential polyunsaturated fatty acids as well as encapsulated water-soluble nutrients.
Recently, lipid spray-beads have been developed that consist of particulate nutrients embedded in lipid spheres. The beads can be prepared by spraying the lipid-nutrient mixture into a cool chamber to harden the lipid, allowing the beads to be collected and stored. The advantage of this method is that higher concentrations of core material can be encapsulated and the preparation process is simpler than for lipidwalled microcapsules.
It is likely that the future microparticles for the effective delivery of all dietary nutrients to marine suspension feeders will consist of lipid beads or capsules containing water-soluble nutrients that are themselves embedded in microbound or microcapsule dietary particles.
This combination of particle types is called a “complex” microparticle (Figs. 3 and 4). Feeding experiments with penaeid shrimp larvae indicate that complex particles can be ingested and dietary contents released in the digestive system. Commercial development of such complex particles should greatly reduce reliance on live feeds and lead to less expensive, dependable, high quality artificial diets for marine suspension-feeders.
(Editor’s Note: This article was originally published in the February 2000 print edition of the Global Aquaculture Advocate.)
Now that you've reached the end of the article ...
… please consider supporting GSA’s mission to advance responsible seafood practices through education, advocacy and third-party assurances. The Advocate aims to document the evolution of responsible seafood practices and share the expansive knowledge of our vast network of contributors.
By becoming a Global Seafood Alliance member, you’re ensuring that all of the pre-competitive work we do through member benefits, resources and events can continue. Individual membership costs just $50 a year.
Not a GSA member? Join us.
Author
-
Chris Langdon
Coastal Oregon Marine
Experiment Station
Hatfield Marine Science Center
Newport, Oregon 97365 USA[117,100,101,46,116,115,114,111,46,99,115,109,104,64,110,111,100,103,110,97,76,46,115,105,114,104,67]
Tagged With
Related Posts
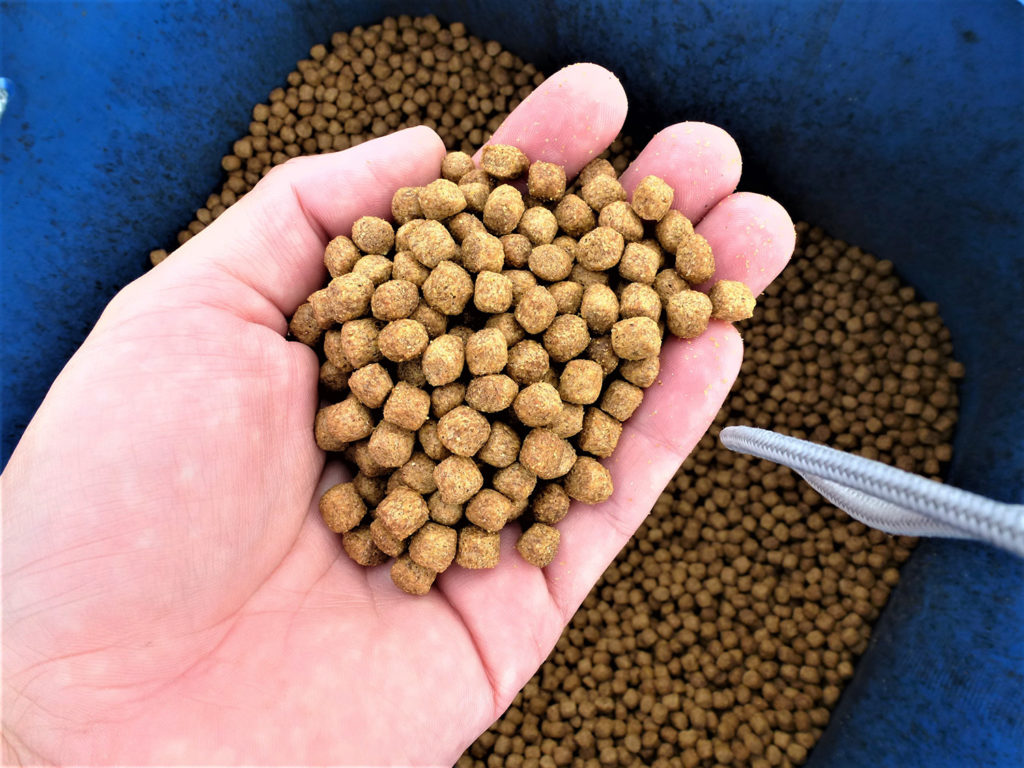
Health & Welfare
Advances in tilapia nutrition, part 2
Nutrition has an important role on growth, performance and flesh quality of tilapia. Part two of this two-part series looks into mineral supplementation and feeding strategy.
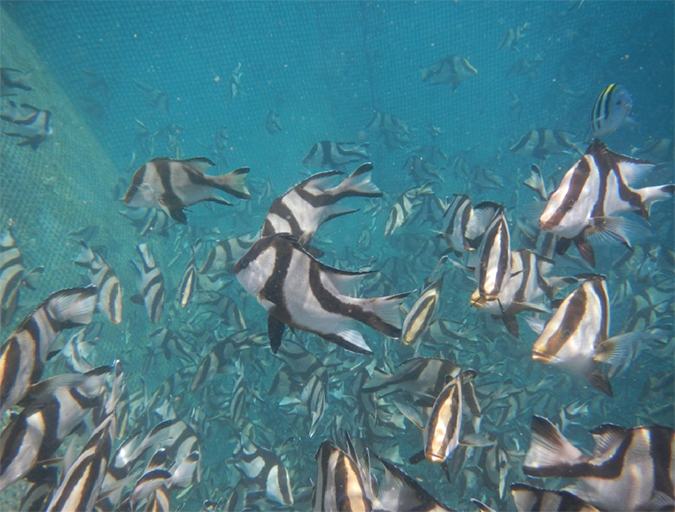
Responsibility
Assessing culture potential of red emperor snapper in New Caledonia
The red emperor snapper, known as “pouatte” in New Caledonia, is valuable throughout its broad geographic range and a highly valued food fish locally. Declining wild catches and market demand have provided the incentive to carry out technical feasibility studies to determine its commercial aquaculture potential.
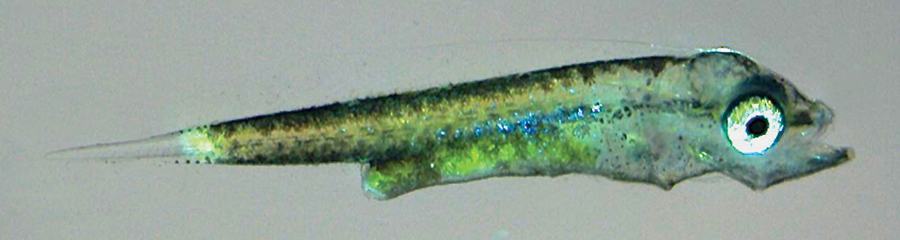
Aquafeeds
Liposomes open new doors in larval fish nutrition
Liposomes, synthetic microparticles originally developed for the pharmaceutical industry, can be produced for ingestion by rotifers and artemia. A study found that yellowtail larvae fed rotifers enhanced with liposomes containing taurine had improved growth.
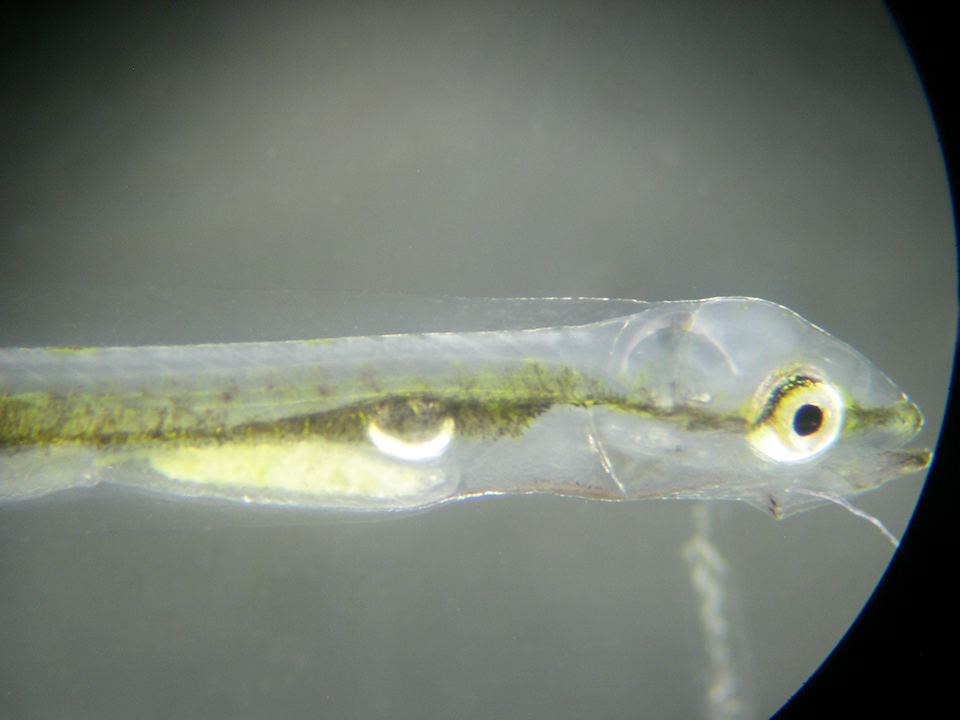
Health & Welfare
Sea bass hatchery feeds artemia substitute to increase production, stability
After experiencing disappointing survival rates following traditional artemia-based rearing protocols, the French hatchery Aquastream turned to a micropellet alternative to artemia.