Knowing the unique characteristics of water is important to quality management in aquaculture
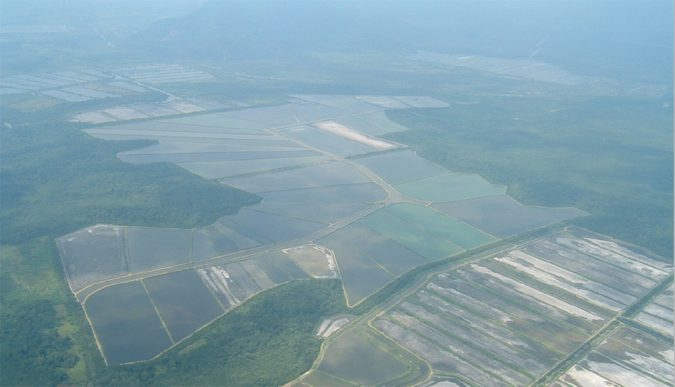
Water is the milieu for aquaculture, and most of my columns have been about water quality and its effects on farmed aquatic animals. The physical properties of water are unique, and greatly affect the behavior of water in nature and in aquaculture systems. Most aquaculturists spend considerable effort maintaining adequate water quality in culture systems. Many may have overlooked or forgotten about the uniqueness of water and how its basic properties influence aquaculture systems.
Pure water at room temperature is a clear, odorless, colorless liquid of simple molecules each consisting of two hydrogen atoms and one oxygen atom. The unique arrangement of these atoms in water impacts a small negative charge to one side of its molecule and a positive charge to two sites on the other side. Positively charged sites on water molecules attract the negatively charged sites of another water molecule to form bonds known as hydrogen bonds.
Because of hydrogen bonding, the strength of attraction among water molecules is much greater than would be expected, and this attraction among water molecules imparts unique – almost magical – properties to water.
Thermal properties
Freezing and boiling points, 0 degrees-C and 100 degrees-C, respectively, of water at normal atmospheric pressure are much higher than those of other hydrogen compounds of low molecular weight. Water freezes when its energy content declines and molecular motion slows so that hydrogen bonds form to produce the crystalline structure of ice. Ice melts when its energy content rises, molecular motion increases, and breaks enough hydrogen bonds to cause the crystalline structure of ice to collapse.
At the freezing point, 80 calories of heat must be removed from or added to each gram of water to cause it to change phase – to freeze or to melt with no change in temperature. Once melted, only 1 calorie per gram of water is needed to increase temperature by 1 degree-C. The heat-holding capacity of water is high compared to other substances found on the landscape.
The spacing of molecules in the crystalline lattice of ice creates a void, and a volume of ice weighs about one-eleventh less than the same volume of liquid water, allowing ice to float. This prevents water bodies from freezing solid in cold climates. The specific gravity of water increases as temperature rises until maximum specific gravity of 1.000 is attained at about 39 degrees-F (4 degrees-C). Further warming decreases the specific gravity of water.
The dissolved mineral content or salinity also influences the specific gravity of water. Cooler water is slightly heavier than warmer water, and seawater is slightly heavier than freshwater. Density (weight/volume) differences caused by temperature allow freshwater bodies to stratify, and salinity differences can cause saline water bodies to stratify.
Water changes from liquid to vapor when it attains enough internal energy and molecular motion to break all hydrogen bonds. Water vapor condenses to form liquid water when it loses energy and molecular motion decreases to permit formation of hydrogen bonds. The amount of energy necessary to change liquid to vapor (or vice versa) at the boiling point is 540 calories per gram.
However, water does not have to reach 100 degrees-C to evaporate. At lower temperatures, water changes from liquid to water vapor because some water molecules have more energy than others and escape the surface to evaporate. This fact does not change the energy requirements for evaporating water.
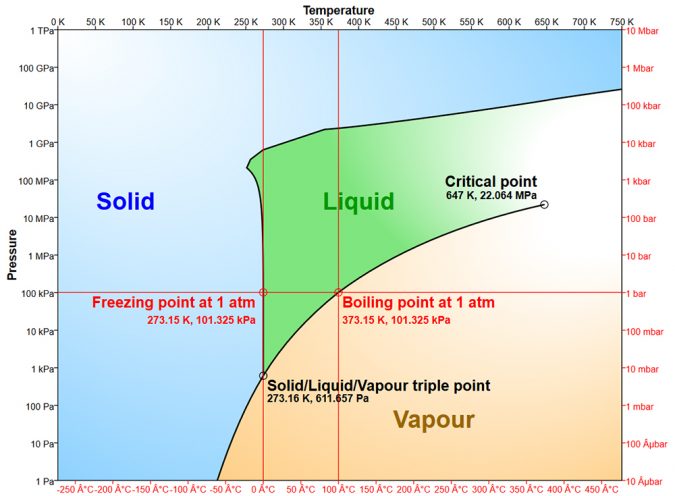
Water vapor
If a surface water is exposed to dry air, water molecules enter the air until it is saturated. The pressure of the water molecules in the air acting down on the water surface is the vapor pressure of water. Vapor pressure increases as temperature rises, and when it becomes equal to atmospheric pressure, bubbles form and push back the atmosphere – the water boils.
The relative humidity of air is the percentage of the moisture holding capacity of air that is filled with water vapor at a particular time. Low relative humidity favors evaporation. Decreasing air temperature will increase relative humidity and vice versa. When air reaches 100 percent relative humidity, condensation of water vapor contained in it will occur. Clouds result when air rises, cools, becomes saturated with water vapor, and condensation occurs. Moisture droplets of clouds may grow until they are heavy enough to fall as rain or frozen precipitation.
Water and surfaces
Water molecules are cohesive when they form hydrogen bonds with each other, but they are adhesive when they form hydrogen bonds with molecules of other substances. Water adheres to a solid surface if its molecules have a greater tendency to form hydrogen bonds with the surface than with other water molecules – such a surface is called hydrophilic because it wets easily.
Cohesive forces between water molecules may tend to be greater than the attraction of water molecules to a solid surface; water will bead from such a surface and not wet it – such a surface is called hydrophobic. For example, raw wood wets readily, but a coat of paint will cause the wood to shed water. If adhesion is greater than cohesion, the surface is hydrophobic
Water molecules at a water surface are subjected to an inward cohesive force from molecules below the surface, but there is no such attraction above the surface. This causes surface molecules to act as a skin, a phenomenon known as surface tension. Surface tension is strong enough to permit certain insects and spiders to walk on the surface of water, and to allow needles and razor blades to float.
As adhesion drags the surface film upward, the surface film – because of cohesion to molecules below – pulls water up the tube against the force of gravity. The height that water rises in a thin tube is inversely proportional to tube diameter. In the soil, open space exists because soil particles do not fit together perfectly. Interconnected pore space in soil functions in the same manner as a capillary tube, permitting water to rise above the water table in a dry soil. Thus, bottom soils in empty ponds where the water table is near the pond bottom may not dry completely.
Water has little or no elasticity of shape and conforms to the shape of its container. Unless completely confined, water has a free surface that is horizontal except at the edges. The pressure of water in a vessel is equal to the weight of the water pressing down on the bottom; thus, in practice water pressure often is given in terms of water depth and called the head.
As everyone knows, water flows downhill, from higher head to lower head. Water has viscosity or internal resistance to flow resulting from cohesion between water molecules, interchange of particles between layers of different velocities, and friction between the fluid and solid surfaces. In laminar flow, water moves in layers with little exchange of molecules among layers. When flow becomes turbulent, the molecules no longer flow in layers and the movement of water becomes more complex.
Water as solvent
Salt crystals maintain their structure in air because of electrical attraction between their component ions. For example, a crystal of table salt – sodium chloride – is comprised of positive sodium ions and negative chloride ions attracted to each other. Salt dissolves readily in water because the attractive forces between negative ions and positive ions are less in water than in air.
Negative ions attract the positive sites on several water molecules, and positive ions attract the negative sites of other water molecules – a process called hydration. This attraction of water molecules insulates the charges on ions and lessens the degree of attraction between them. Thus, water is an excellent solvent for most inorganic salts and many organic substances.
Conductivity is the ability of a substance to convey an electrical current. In water, electrical current is conveyed by the dissolved ions. Pure water does not conduct electricity well, because it contains only a few hydrogen and hydroxide ions.
Natural waters, however, contain more dissolved ions, and they conduct electricity very well. Conductivity increases in direct proportion to dissolved ion concentrations, and provides a simple way of estimating the concentrations of ions (salinity of water). This measurement is often used in aquaculture.
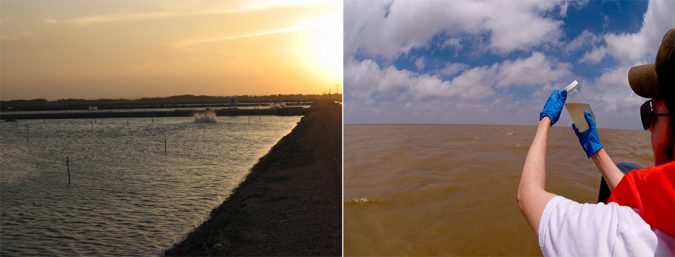
Light and turbidity
Part of the sunlight striking natural water bodies does not penetrate the surface. A portion of the light is reflected, the amount depending upon the roughness of the water surface and the angle of the sun’s rays. The smoother the surface and the nearer to vertical of the sun’s rays, the greater is the percentage of light penetrating the water surface.
In pure water, roughly 50 percent of incident light is transformed into heat within the first meter of depth. Natural waters contain substances that further interfere with light penetration. The color of natural water results from the unabsorbed light rays remaining from the original light. Substances in solution or in colloidal suspension cause true color in water.
Apparent color is caused by suspended matter which interferes with light penetration. During the day, photosynthesis by aquatic plants increases as light increases and decreases as light wanes. Clouds can reduce the amount of sunlight striking water surfaces and immediately lower the rate of photosynthesis.
Turbidity refers to the decreased ability of water to transmit light caused by suspended particulate matter – usually soil particles, minute living plants and animals (plankton), and particles of decaying plankton or other organic remains. A certain amount of turbidity caused by phytoplankton is desirable in aquaculture ponds because it prevents light from striking the bottom and encourages underwater weeds. High turbidity concentration from suspended clay particles lessens photosynthesis rate.
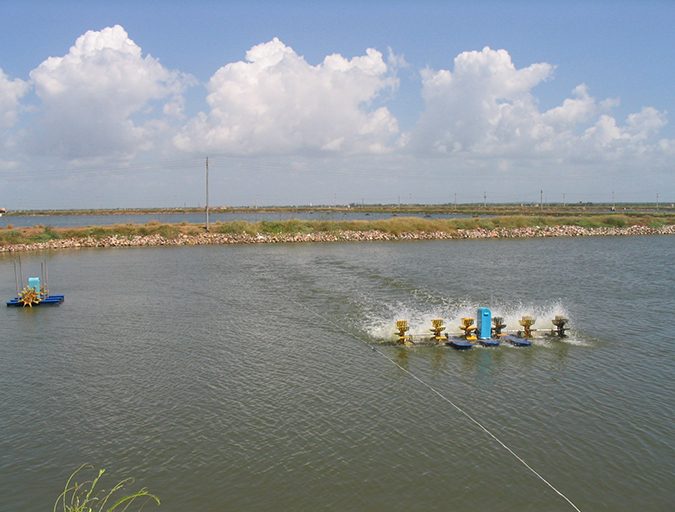
Thermal stratification
Water temperature closely follows air temperature in ponds, and changes markedly with season. At the end of winter, the water column in a lake or pond has a relatively uniform temperature and density. Although heat is absorbed at the surface on sunny days, there is little resistance to mixing by wind and the entire volume of the water body circulates and warms uniformly. As spring progresses, the upper stratum heats more rapidly than heat is distributed from the upper stratum to the lower stratum by mixing.
Waters of the upper stratum become considerably warmer and lighter than those of the lower stratum. If the pond is more than 2 to 3 meters maximum depth, winds that normally decrease in velocity as weather warms no longer are powerful enough to mix the two strata because of the difference in density.
The upper stratum is called the epilimnion and the lower stratum the hypolimnion. The stratum between the epilimnion and the hypolimnion has a marked temperature differential. This layer is termed the thermocline. In the tropics, deep ponds usually stratify in the dry season and destratify in the wet season, or they may remain stratified year around.
Aquaculture ponds should not be deeper than 2 meters to avoid thermal stratification. Of course, mechanical aeration mixes pond water and can prevent stratification even in somewhat deeper ponds.
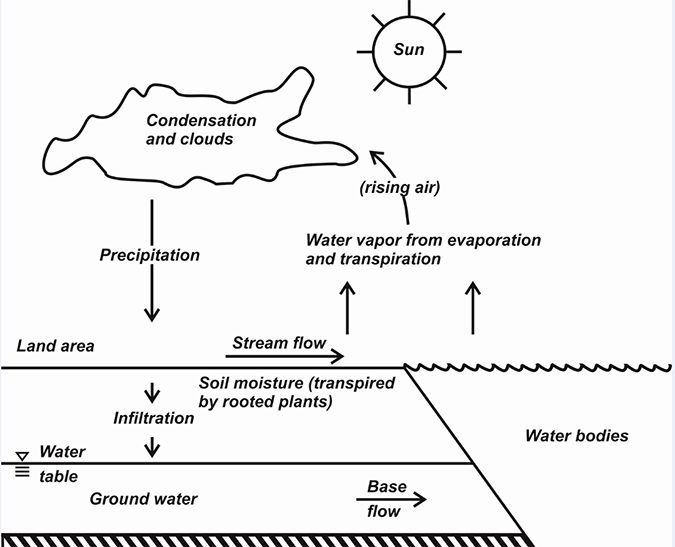
The hydrologic cycle
The familiar and continuous motion of the earth’s water in the hydrologic cycle is depicted in Fig. 3. This cycle has long been recognized. A passage of the Old Testament refers to the rivers flowing into the ocean but the ocean not being full. Water evaporates from the ocean, lakes, ponds, and streams and from moist soil; water also is transpired by plants.
The sun provides energy to change liquid water to water vapor, so solar radiation is the engine that drives the hydrologic cycle. Water vapor is caught up in the general atmospheric circulation, and in places where there is uplifting and cooling of air, water vapor condenses to solid or liquid water and is returned to the earth’s surface as dew, frost, sleet, snow, hail, or rain.
A portion of the water reaching the earth’s surface evaporates back into the atmosphere almost immediately, but another part runs over the land surface as storm runoff, collects in streams, rivers, and lakes, and finally enters the ocean. Water is continually evaporating as the runoff flows toward the oceans, and it continually evaporates from the ocean.
Some water infiltrates into the ground and becomes soil water or reaches saturated strata to enter groundwater aquifers. Soil water can be returned to the atmosphere by transpiration of plants. Groundwater seeps into streams, lakes, and the ocean, and it may be removed by wells for use by mankind – frequently for aquaculture.
Seepage and evaporation from ponds determine the amount of water that must be added to maintain water levels in ponds. Also, if ponds are rainfed, watershed runoff is the water source, and the ratio of watershed area to pond volume and seepage and evaporation rates is critical. Thus, aquaculturists should be familiar with the local hydrological cycles and its variation among seasons and years.
Author
-
Claude E. Boyd, Ph.D.
School of Fisheries, Aquaculture and Aquatic Sciences
Auburn University
Auburn, AL 36830 USA[117,100,101,46,110,114,117,98,117,97,64,49,101,99,100,121,111,98]
Tagged With
Related Posts
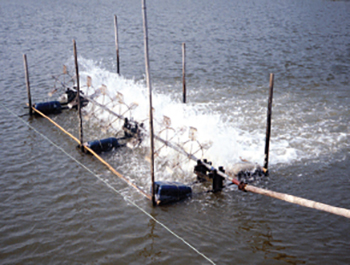
Responsibility
Efficiency of mechanical aeration
Although relatively expensive, mechanical aeration increases production. Asian-style paddlewheel aerators are widely used but are inefficient. Testing has led to more efficient designs that are now widely used in U.S. catfish and shrimp ponds.
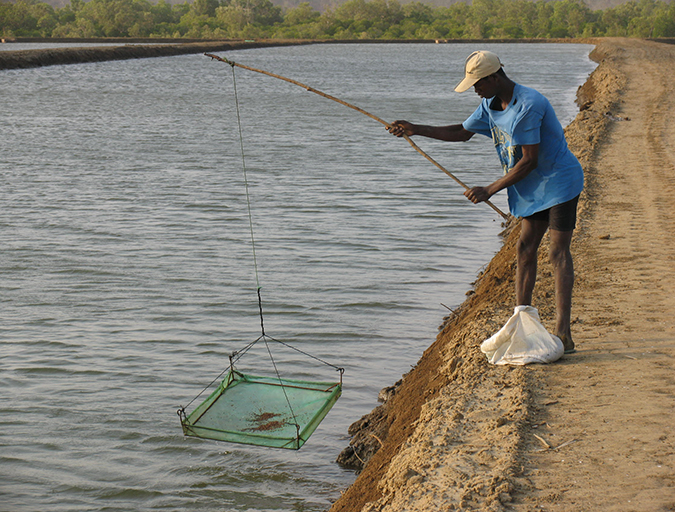
Health & Welfare
Decomposition and accumulation of organic matter in ponds
Aquaculture ponds accumulate organic matter from organic fertilizer, remains of microorganisms produced within the pond, feces of the culture animals and uneaten feed. Claude E. Boyd, Ph.D., details the leading organic matter management practices, and says that the accumulation of organic matter is often not as great as believed.
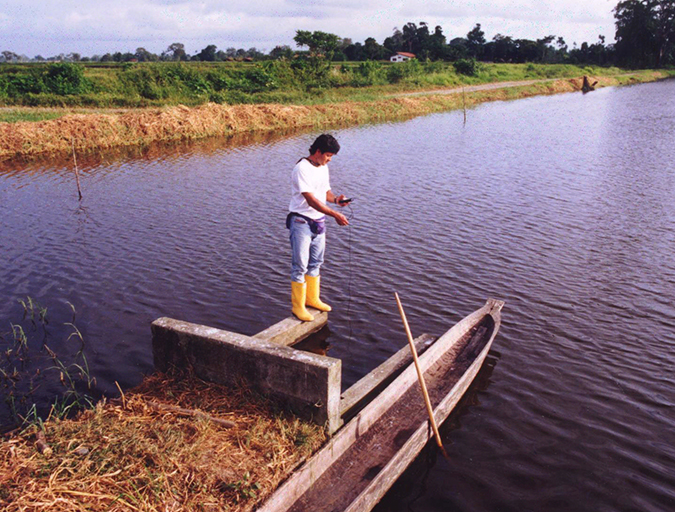
Responsibility
The importance of water analysis in aquaculture
Proper monitoring of water quality in aquaculture production systems is critical to enable appropriate and timely management decisions. It requires reliable equipment, trained technicians that follow instructions and apply quality control measures, proper reagents and calibrated equipment, and appropriately collected water samples that are promptly analyzed.
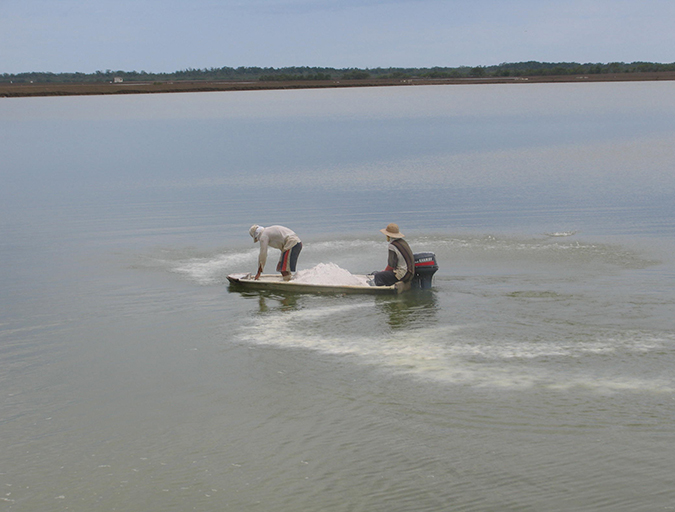
Responsibility
What causes alkalinity changes in aquaculture waters?
Total alkalinity is an important variable in water for aquaculture systems, and its concentration frequently fluctuates over time in many culture systems.