Study shows composition altered by nutritional biorhythms
The intestinal microbiota has been linked to a wide range of biological processes that benefit the host, including nutritional conditions and the immune system. Microbiota composition drastically changes in response to environmental and biological conditions. The alternation of microbiota composition affects nutritional absorption and regulation of host energy balance, and interactions between host and gut microbiota caused by dietary conditions have been reported in many animals including fish. Moreover, the feeding and fasting cycle involves both host metabolism and gut microbiome composition and could lead to obesity and metabolic diseases.
The gut microbiota has also been shown to change according to the circadian rhythm (a natural, internal process regulating the sleep-wake cycle, repeating about every 24 hours) because of the link with the host circadian rhythm. Knowledge regarding the gut microbiota of teleostean (ray-finned) fishes has been gradually accumulating; however, information regarding dynamics and interaction with host fish remains limited and appears to differ according to condition and species.
This article – adapted and summarized from the original publication – reports on a study to characterize the composition of the gut microbiome of the leopard coral grouper and to investigate microbiome dynamics from fasting conditions to feeding conditions. The microbiota functional capacity was subsequently estimated and compared to the host transcriptome (the set of all RNA molecules in one cell or a population of cells) and metabolome (the complete set of small-molecule chemicals found within a biological sample) data. Our analysis suggests organization of the leopard coral grouper host metabolism and its microbiota metabolism.
Study setup
Eight-month-old leopard coral grouper (each approx. 60 grams in weight) were maintained in 60,000-liter tanks with a flow-through system at the Yaeyama Laboratory, Seikai National Fisheries Research Institute, Fisheries Research Agency in Japan. The facility was illuminated by sunlight with a natural photoperiod (11 L:13 D) during the winter. The fish were fasted for the first two days of the experiment and were then fed [Zeitgeber time (ZT) 2 and ZT10] to satiation for the next two experimental days.
Fish were fed a commercial fish feed (Higashimaru Co., LTD.; Kagoshima, Japan) with >46 percent protein, >10 percent fat, <15 percent carbohydrates, <2.5 percent fiber, >2.0 percent calcium and >1.0 percent phosphorus.
Gut contents were collected every 4 hours, except during the night of day 1 and day 3. At each sampling point, three to twelve fish were sampled individually. Seawater samples were collected from outside of the facility (costal seawater), the inlet, the rearing tank, and the outlet every 12 hours (ZT2 and ZT12). Skin mucus samples were collected from six fish at ZT10 of day 2 (fasting) and day 4 (feeding). Samples were collected and stored frozen until used for experiments.
For detailed information on fish husbandry and sampling, DNA extraction and DNA library construction, Sequencing and data analysis, please consult the original publication.
Results and discussion
The microbiota community was investigated under fasting and feeding conditions. Proteobacteria was the dominant phylum during fasting (Fig 1A), as well as in the seawater in the rearing tanks. Subsequent to feeding, Firmicutes became the dominant phylum. As fish were fed at ZT2 and ZT18 from day 3 and the dominant phylum had changed by ZT14 at day 3, it took approximately 12 hours for the dominant phyla to interchange.
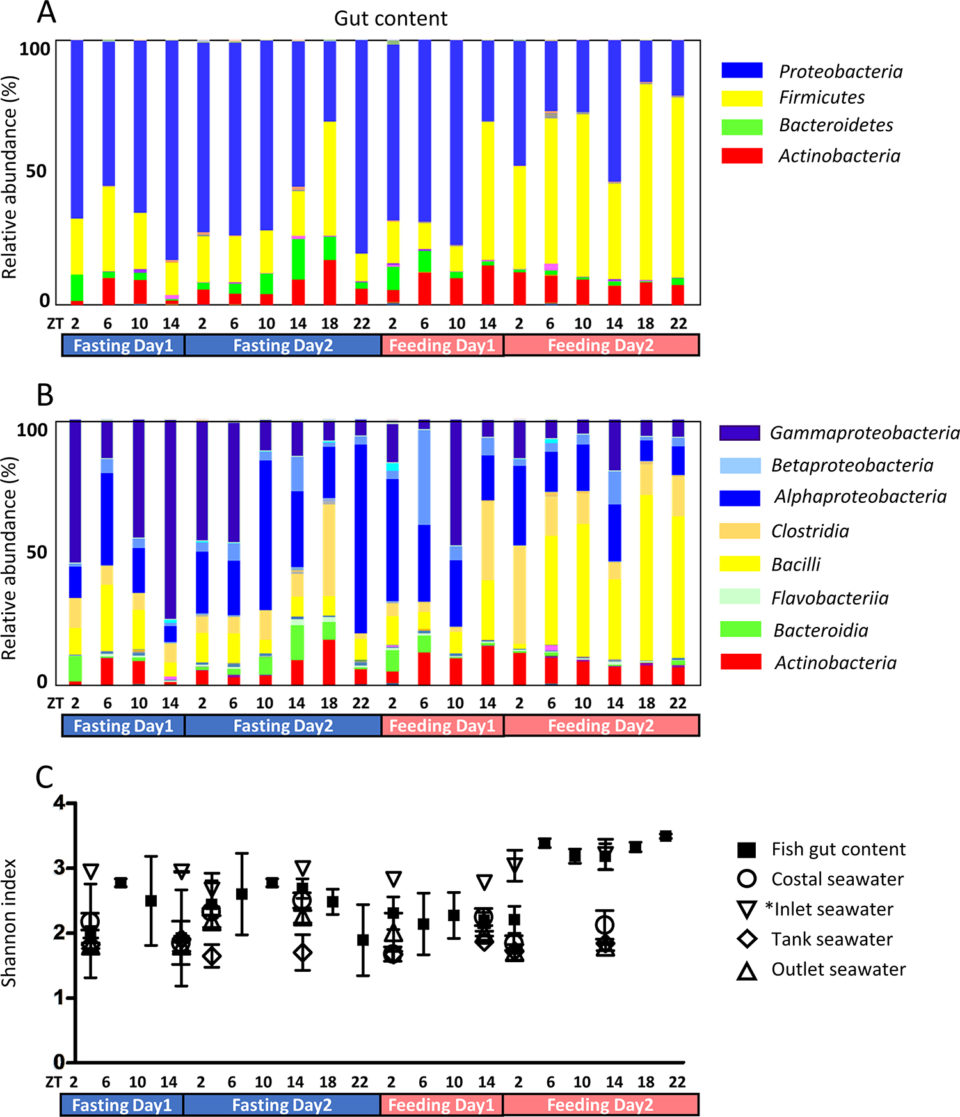
During fasting, Gammaproteobacteria were dominant, most likely originating from ingested seawater (Fig 1B). Fish drink seawater to compensate for dehydration. In most cases, Gammaproteobacteria have been reported to be dominant in rearing tanks; the skin and gut of fish from both the sea and rearing tanks are rich in Gammaproteobacteria.
In our study, Gammaproteobacteria was the most dominant class in the rearing tank seawater and skin mucus and the proportion of Firmicutes and Actinobacteria was higher in inlet seawater than in other seawater. As inlet seawater was subjected to UV sterilization, microbiota composition appears to have changed. Microbiota abundance was also significantly low due to sterilization. The microbiota in fish gut changed after feeding (ZT14 of day 3) and the composition of microbiota in rearing tank seawater changed simultaneously, suggesting that tank seawater is affected by fish feeding conditions.
Skin mucus microbiota was also investigated (Fig. 2). The fasting gut content consists almost entirely of mucus; therefore, the skin mucus microbiota and the fasting gut microbiota were similar. Gut microbiota biodiversity was higher after day 2 of feeding compared to the other experimental time points (Fig 1C). In seawater samples, biodiversity did not change during the experiment, while skin mucus biodiversity slightly decreased during the fasting period. Feeding conditions might affect not only the digestive tract, but the entire body.
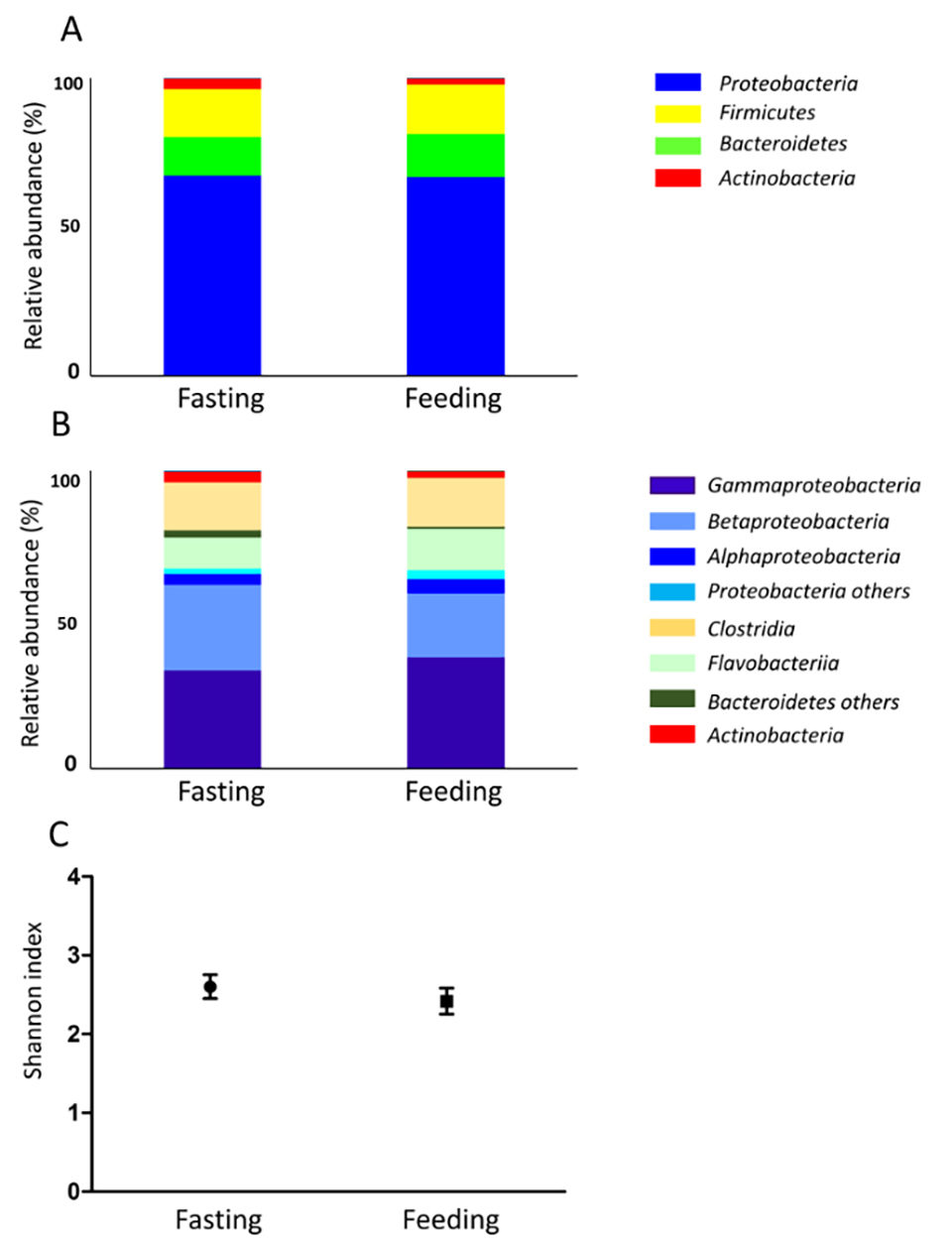
As microbiota composition changes in response to feeding and fasting conditions, it might also affect the host nutritional condition and it is hypothesized that the gut microbiota interacts with the host fish. The alternation of microbiota composition affects nutritional absorption and regulation of host energy balance. Although gut microbiota did not exhibit diurnal oscillation in our study, our results show that fish microbiota composition changed within two days according to nutritional conditions.
Phylogenetic diversity changed according to feeding conditions (Fig 1C). The index was elevated after F4-ZT6 (feeding day 2, ZT 6), while the bacterial population increased after approximately 24 hours. A similar tendency of phylogenetic diversity under fasting conditions has been observed in tilapia. Phylogenetic diversity has also been reported to change between wild and farmed fish. Compared to the gut content, the phylogenetic diversity of seawater was low. Gut microbiota is thought to be complex in order to fulfill symbiosis functions.
Hierarchical cluster analysis (HCA; an algorithm that groups similar objects into groups called clusters) revealed three clusters: 1) high during fasting, 2) high during feeding, and 3) others. Proteobacteria and Bacteroidetes belong to the fasting group, while Firmicutes and Fusobacteria belong to the feeding group.
Subsequent correlation analysis revealed that Firmicutes and Fusobacteria exhibited a positive correlation, while Firmicutes and Bacteroidetes showed a negative correlation. It has been widely established that Firmicutes increase under conditions of high fat feed intake and that Firmicutes and Bacteroidetes exhibit a negative correlation. Although these reports examined mammals, fish have also exhibited a similar relationship between Firmicutes and Bacteroidetes. Our previous study showed the farmed fish store excess amounts of visceral fat. This excess amount of fat is thought to originate from feed; therefore, the number of Firmicutes is predicted to increase.
We investigated the dynamics of gut microbiota from fasting to feeding. Dominant bacteria during fasting are predicted to originate from ambient seawater. Following feeding, microbiota composition gradually changed and reached the feeding condition type 12 hours from the start of feeding. Our analysis identified the following key bacteria: Firmicutes and Fusobacteria for feeding conditions, and Proteobacteria for fasting conditions. Gammaproteobacteria was the dominant Proteobacteria class in ambient seawater, which is different from natural seawater. Gammaproteobacteria have been reported to be enriched in fish mucus, skin, and intestine; therefore, the Gammaproteobacteria in ambient seawater most likely originate from fish.
In addition, our comparison of microbiome data with transcriptome and metabolome data indicated microbial fluctuation following nutritional input is more significant than host metabolism. Finally, comprehensive analysis of the microbiome and host metabolism could identify key factors for monitoring aquaculture environment and symbiotic metabolism upon feeding (Fig 3).
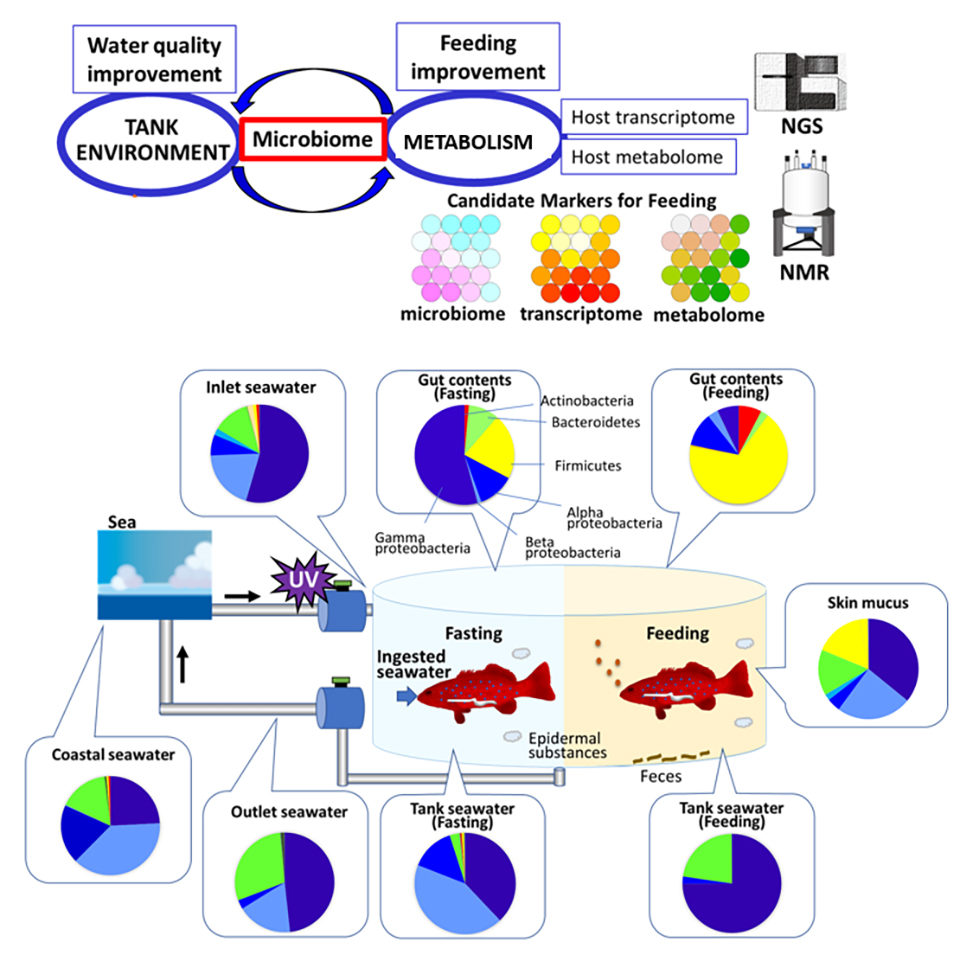
Perspectives
Results of our study showed that microbiota composition changed in response to feeding and fasting conditions and could affect nutritional control and energy balance. The feeding and fasting cycle involves host metabolism and is linked to obesity and metabolic diseases. In this study, the composition of fish microbiota dramatically changed within two days of feeding and fasting cycles. As gut microbiota influence host fish metabolism, elucidating the relationship between gut microbiota and host fish might prove crucial for successful and efficient production of cultured fish.
The fish microbiota can indicate the condition of the fish metabolism and immune systems. Environmental seawater directly affects fish conditions; therefore, monitoring microbiota in ambient seawater is also important for maintaining fish health. Controlling microbiota constitutes one approach for ensuring successful aquaculture.
Now that you've reached the end of the article ...
… please consider supporting GSA’s mission to advance responsible seafood practices through education, advocacy and third-party assurances. The Advocate aims to document the evolution of responsible seafood practices and share the expansive knowledge of our vast network of contributors.
By becoming a Global Seafood Alliance member, you’re ensuring that all of the pre-competitive work we do through member benefits, resources and events can continue. Individual membership costs just $50 a year.
Not a GSA member? Join us.
Authors
-
Miyuki Mekuchi
RIKEN Center for Sustainable Resource Science
Tsurumi-ku, Yokohama, Kanagawa, Japan;
and National Fishery Research Institute of Fishery Sciences
Fishery Research and Education Organization
Kanazawa-ku, Yokohama, Japan -
Taiga Asakura, Ph.D.
RIKEN Center for Sustainable Resource Science
Tsurumi-ku, Yokohama, Kanagawa, Japan -
Kenji Sakata
RIKEN Center for Sustainable Resource Science
Tsurumi-ku, Yokohama, Kanagawa, Japan -
Dr. Tomofumi Yamaguchi
Research Center for Subtropical Fisheries
Ishigaki, Japan -
Dr. Kazuhisa Teruya
Research Center for Subtropical Fisheries
Ishigaki, Japan -
Jun Kikuchi, Ph.D.
Corresponding author
RIKEN Center for Sustainable Resource Science, Tsurumi-ku, Yokohama, Kanagawa, Japan;
and Graduate School of Medical Life Science, Yokohama City University, Tsurumi-ku, Yokohama, Kanagawa, Japan;
and Graduate School of Bioagricultural Sciences, Nagoya University, Chikusa-ku, Nagoya, Aichi, Japan[112,106,46,110,101,107,105,114,64,105,104,99,117,107,105,107,46,110,117,106]
Tagged With
Related Posts
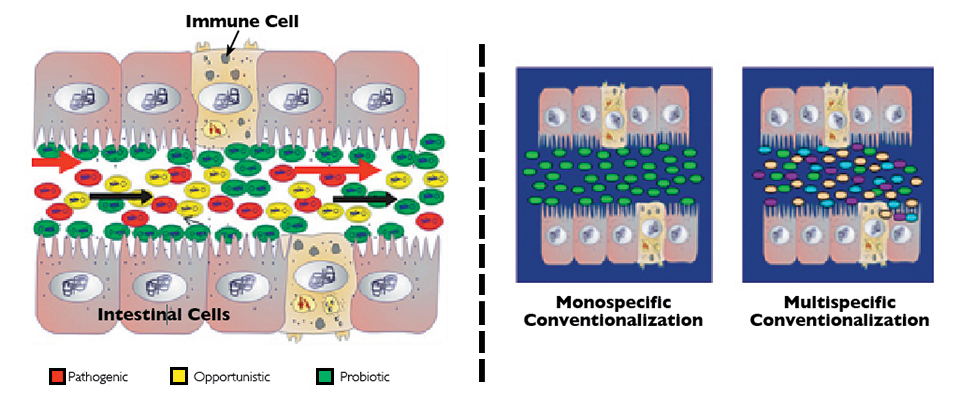
Health & Welfare
Domestication of gut microbiota can improve shrimp aquaculture
In studies, bacteria and yeasts isolated from the native microbiota of wild shrimp at larval, juvenile and adult life stages were administered to early-stage shrimp via the culture water.
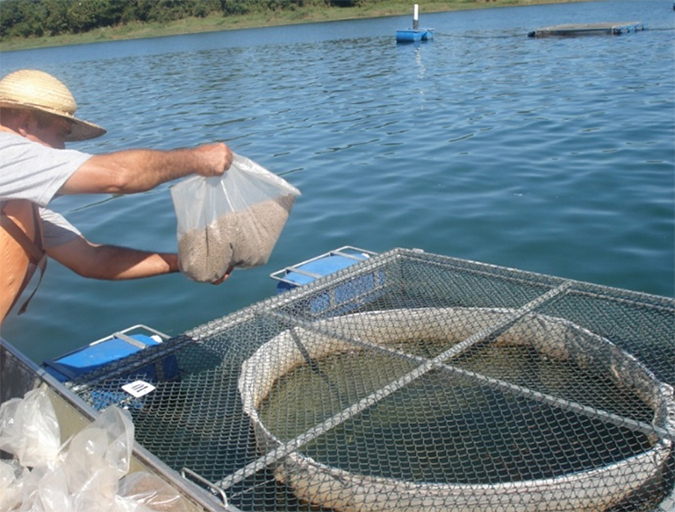
Health & Welfare
Aiding gut health with a natural growth promotor
A study with Nile tilapia conducted in commercial production cages in Brazil showed the potential – in the absence of major disease threats – of a commercial, natural growth promotor that modulates the microbiota (inhibiting growth of pathogenic bacteria and promoting growth of beneficial bacteria) and inhibits quorum sensing.
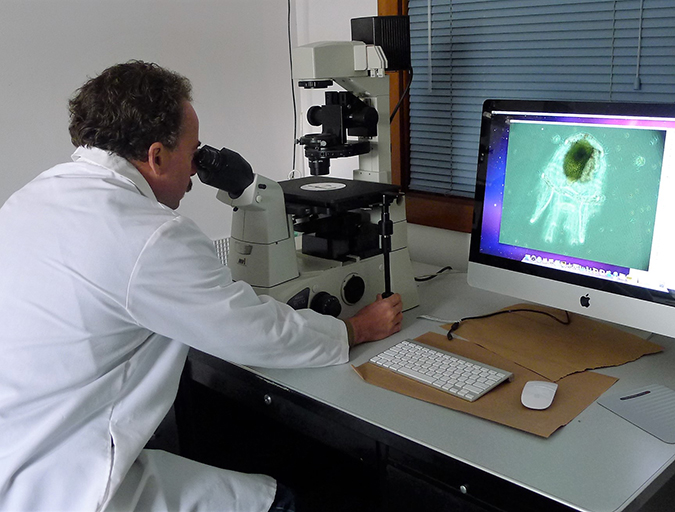
Innovation & Investment
Better together: Partnerships drive innovation at leading labs
Laboratories with industry partnerships are making aquaculture more innovative, efficient and responsible. These collaborations offer access to expertise, facilities and funding to further the industry and improve global food security.
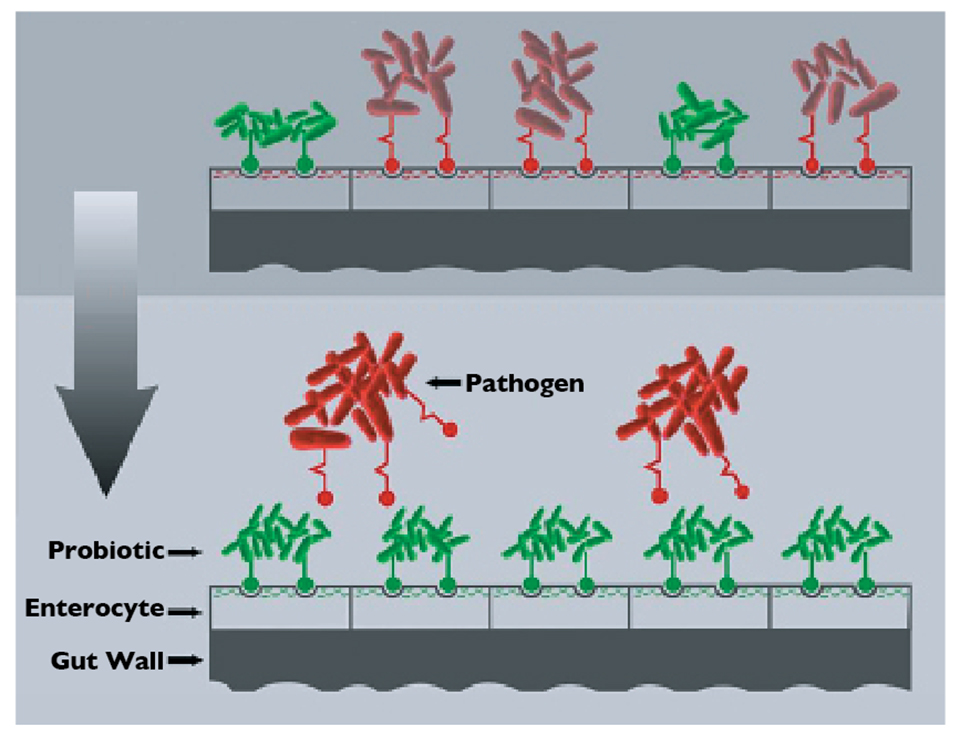
Aquafeeds
Varied feed additives improve gut, animal health
There is increasing evidence that natural feed additives can have beneficial effects on aquaculture animals by supporting well-balanced gut microflora and improving gut health.