Evidence for links between abiotic environmental conditions and WSD outbreaks reviewed: temperature and salinity
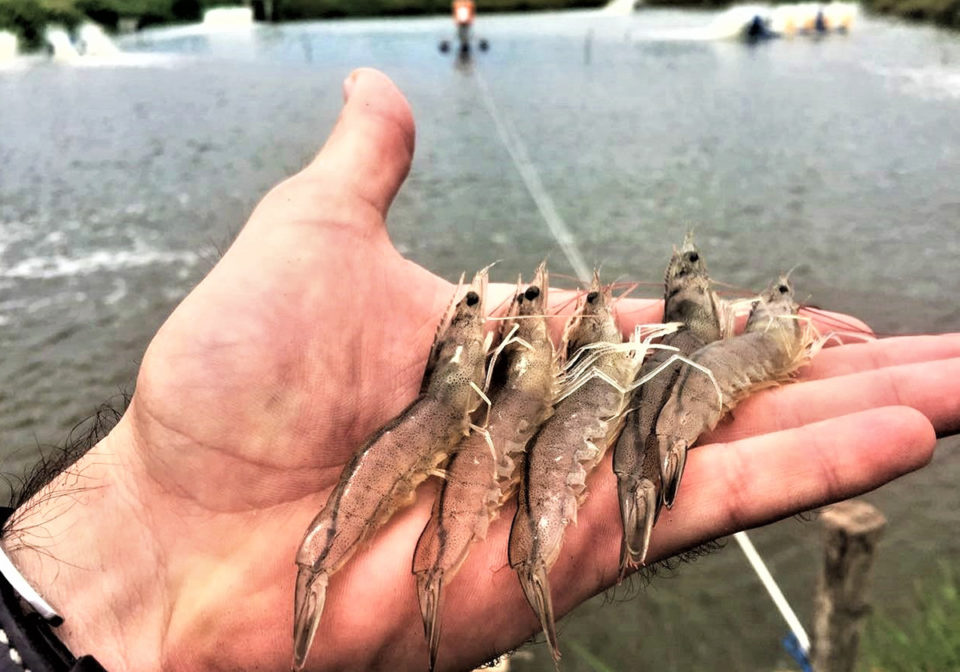
Six viral diseases of crustacea (including White Spot Disease, YWSD; Yellow Head Disease, YHD; and Taura Syndrome, TS) are currently listed by the World Organisation for Animal Health (OIE), recognizing their potential to cause substantial economic loss and spread to shrimp farming and trading countries. Of these, the greatest threat to shrimp aquaculture worldwide is WSD, caused by the White Spot Syndrome Virus (WSSV). This single disease has reportedly caused an estimated (U.S.) $21 billion in economic damage since its emergence was detected in 1992.
WSSV infection affects both wild and farmed shrimp populations, but its impacts are most significant within intensive aquaculture settings, where animals are reared at unnaturally high densities. Most reviews thus far have focused on host physiology and molecular responses to infection, nutrition and feed enhancement, and the application of probiotics to prevent disease outbreaks. However, WSD outbreaks are increasingly associated with changes in environmental conditions that may lead to physiological stress and a compromised ability of shrimp to resist disease. Changes in water temperature, salinity, oxygen levels and pH are particularly important to ensure good animal health in aquaculture systems and managing water quality remains a challenge for many shrimp farms.
It is important that the relationship between disease susceptibility and environmental abiotic conditions are determined, as suboptimal conditions within shrimp farms may promote infection, leading to increased losses across farms. Moreover, there may be practical solutions relating to improvements in specific pond conditions that could serve to reduce the rates of shrimp diseases.
This article – adapted and summarized from the original publication (Millard, R.S. et al. 2020. How do abiotic environmental conditions influence shrimp susceptibility to disease? A critical analysis focused on White Spot Disease. Journal of Invertebrate Pathology, April 2020) – reports on a comprehensive review that critically evaluated evidence from the literature on the effects of alterations in abiotic environmental conditions on the occurrence and severity of WSD outbreaks.
https://www.aquaculturealliance.org/advocate/shrimp-farming-industry-in-ecuador-part-1/
Study setup
For each abiotic parameter considered in this review, we summarized the available information on their effects on penaeid shrimp physiology, evaluated evidence for altered WSD outbreak occurrence or severity and discussed some of the mechanisms that may lead to altered susceptibility (e.g., compromised immune response).
In this review we also considered the potential for conditions in aquaculture systems to be optimized for disease prevention, given their importance as predictors of outbreaks. And we additionally identified areas in which further research will facilitate our understanding of disease dynamics and implementation of practical solutions to the problems caused by WSD in an aquaculture setting.
Detailed information on the literature reviewed on WSD, WSSV temperature, salinity, dissolved oxygen concentration, hypercapnia [buildup of carbon dioxide in the body of an organism] and pH, and nitrogenous compounds; and their relevance to shrimp farming, refer to the original publication.
Temperature
Shrimp are typically reared in enclosed, shallow ponds where low levels of water exchange coupled with tropical weather conditions allow for significant seasonal and diurnal fluctuations in water temperature to occur. The most commonly farmed penaeid shrimp, the Pacific white shrimp (Litopenaeus vannamei), is able to tolerate wide variations in temperature, ranging from 7.5 to 42.0 degrees-C. The optimal temperature for growth of this species is reported to depend on size, with small shrimp (<5 grams) growing most rapidly at 30 degrees-C and larger shrimp (>16 grams) growing most rapidly at >27 degrees-C.
Many farmers have the capacity to measure temperature conditions within shrimp ponds and how they fluctuate; however, this information is typically not openly available. There are published studies that link rapid temperature fluctuations in ponds (changes of a magnitude of 4.2 to 4.5 degrees-C) following a tropical storm with variation in viral loads and disease outbreak occurrence.
Associations between water temperature fluctuations and WSD outbreaks have been inferred within some studies but no causal link proven. Daily fluctuations of more than 3 to 4 degrees-C have been correlated with episodes of WSD outbreaks. During the monsoon season, where runoff water from tropical storms leads to reductions in pond temperature, outbreaks have been reported. Modelling of historic data suggests that the incidence of WSD is increased in low ambient temperatures (24.5 to 27.2 degrees-C) and where daily atmospheric temperature variations are greater than 10 degrees-C. It is unclear to what extent these outbreaks were initiated by changes in water temperature, or by dilution of salinity and increased contaminants originating from runoff water. Collectively, available studies illustrate the wide natural variation in pond water temperature and a link between unstable temperature conditions and increased WSD outbreaks.
Disease restrictions at high and low temperatures
WSSV has a wide thermal tolerance that overlaps the entire thermal range of L. vannamei, even remaining infective following cryopreservation. It is inactivated upon exposure to temperatures above 50 degrees-C for at least 120 minutes.
The relationship between water temperature and both shrimp growth and WSSV replication is shown in Fig. 1. Controlled laboratory experiments have demonstrated that a temperature range between 25 to 28 degrees-C provides optimum conditions for WSSV infection, resulting in higher levels of mortality, with earlier disease onset and higher WSSV copy numbers in WSSV-infected shrimp (and also reportedly in crayfish). Protection (i.e. reduced mortality and virus replication) from WSD has consistently been reported at high temperatures (>30 degrees-C) regardless of virus exposure route. Very high temperatures (>50 degrees-C) inactivate WSSV but cannot be applied as a WSD control mechanism as they fall well above the maximum thermal tolerance of shrimp.

Laboratory experiments by various researchers have demonstrated that the protective effect of high culture temperatures is dependent on both the duration of the periods of increased temperature and the stage of infection when they occur. In one study with L. vannamei juveniles exposed to WSSV during the acute infection stage (12 hours post-injection), exposure to 33 degrees-C for periods of more than six hours daily delayed signs of outbreak and mortality for both high and low virulence WSSV strains. Conversely, periods of increased temperature that lasted only six hours resulted in accelerated disease progression and increased cumulative mortality.
In a second study, temperature increases from 27 degrees-C to a constant 33 degrees-C during acute infection (prior to clinical signs), reduced and delayed mortality, whereas during chronic infection disease progression was accelerated. During chronic infection, as shrimp succumb to disease, increased stress may prevent the protective effect of high temperature and lead to increased virus replication. However, neither study quantified the viral load to confirm that this effect was due to reduced viral replication, and thus the mechanism that reduces efficacy of increased temperature during chronic infection was not established.
At temperatures of 32 to 33 degrees-C, WSSV reportedly retains the ability to infect and kill L. vannamei. The protective effects seen are therefore not due to viral inactivation and may occur (at least in part) as a result of reduced viral replication. A temperature-induced reduction in viral replication has been demonstrated in a range of invertebrate viruses exposed to high environmental temperatures within their host. These elevated temperatures may be impairing the activity of viral enzymes or enhancing the ability of hosts to respond to infection, with similar mechanisms offering a useful line of investigation in the case of WSSV.
However, the emergence of Taura Syndrome Virus (TSV) isolates from Belize (TSV-BZ) able to replicate under hyperthermic conditions, where the reference TSV strain (TSV-HI) could not, indicates that hyperthermic protection can be strain-dependent, and thermal manipulation as a management technique may result in the appearance of temperature-permissible mutants. Moderate pond temperature increases to >30 degrees-C have also been shown to result in mortalities in uninfected shrimp, reportedly questioning the ubiquity of water temperature manipulation as a suitable solution for preventing infection.
Mechanism of hyperthermic protection
Hyperthermic protection (moving to areas of elevated temperature) is a characteristic feature of several shrimp species to viral infections, such as Infectious Myonecrosis Virus (IMNV) and Infectious Hypodermal and Haematopoietic Necrosis Virus (IHHNV). This suggests that such a strategy may be a ubiquitous response of shrimp to viral infections; however, it is unclear what impact this has on immune function. The presence of such a response has direct implications for enhanced pond design and should be studied.
The mechanisms mediating WSD protection at elevated temperatures are not fully understood. Several studies have proposed that shrimp have increased immunocompetence [capacity for a normal immune response] at elevated temperatures. For example, WSSV-infected shrimp reared at >31 degrees-C had a significantly higher total hemocyte count, THC [blood cells per mL] and phenoloxidase (PO) activity (an integral enzyme involved in invertebrate innate immune defenses) when compared to infected shrimp reared at 27 to 28 degrees-C. Temperature increases may enhance the shrimp’s immune system, but more research is needed.
Temperature management and future directions
Taken together, the literature indicates that the optimum temperature range for virus replication is within the optimal growth range of its penaeid host. This is to be expected, as viruses depend almost entirely on their hosts for their replication. Hyperthermic protection depends both on the duration of hyperthermia and on the stage of infection and occurs by mechanisms that are not yet fully understood but may involve the increased immune responses of the host and/or upregulation of heat shock proteins [family of proteins produced by cells in response to exposure to stressful conditions]. Alterations in temperature have the potential to contribute to the mitigation of WSD but not to completely prevent or treat this disease.
Salinity
Penaeid shrimp are euryhaline [able to adapt to a wide range of salinities] and can generally maintain an internal balance between water and dissolved materials in varying environmental conditions and depending on life stage. Most exchanges of water and ions in penaeids take place in their gill, gut and hypodermal [under the epidermis] tissues and L. vannamei is able to tolerate extreme salinities of 0.5 to beyond 45 ppt.
Salinity has been extensively studied in relation to WSSV infections, as it affects many aspects of shrimp physiology, including metabolic rate, susceptibility to toxic metabolites, feeding rate, molting, growth and development. Salinity is shown to vary greatly in commercial shrimp ponds, typically ranging from 0 to 40 ppt depending on geographic location and is determined by the site-specific degree of water evaporation and rainfall [and also pond water exchange]. L. vannamei naturally inhabits a wide range of salinities from 1 to 40 ppt, with an optimum salinity that is close to its iso-osmotic point [having the same concentration of water as whatever it is being compared to] at 24.7 ppt.
Direct comparison of pond salinity data across sites can be difficult because measurements are not standardized. Pond salinity predominantly depends on the source of water each farm uses, which dictates whether high (seawater) or low (freshwater) salinity culture is employed. In each condition, shrimp may be under osmotic stress during periods when salinity deviates from its optimum, potentially increasing their susceptibility to other stressors and/or disease.
Following periods of heavy rainfall, rapid changes in salinity are likely to result in physiological stress to cultured shrimp and affect their susceptibility to disease. Outbreaks in ponds are frequently recorded following periods of heavy rainfall where salinity drops to below 15 ppt and following heavy surface runoff into ponds. As well as reducing salinity and temperature, such runoff can also carry contaminants that can modify the biotic and abiotic conditions within the ponds.
High and low salinities are linked to disease outbreak
Following WSSV infection, the ability of L. vannamei to osmoregulate [maintenance by an organism of an internal balance between water and dissolved materials regardless of environmental conditions] decreases significantly. Studies have shown that penaeid shrimp maintained at suboptimal salinities were more susceptible to WSD, succumbing to disease earlier, exhibiting increased mortality and displaying increased copy numbers of specific viral genetic material. These effects were most severe when there was a rapid rate of salinity change.
The ability to successfully farm shrimp at stable, low salinities (<5 ppt) is evidence that it is the rate of change, rather than the absolute salinity, that may play a pertinent role in determining disease susceptibility. This is particularly important in the face of climate change, which is projected to result in increased occurrence of extreme weather events that result in rapid alterations to abiotic factors. At high salinities (well above 35 ppt), shrimp have been shown to undergo weight loss and exhibit abnormally low locomotive activity in both infected and uninfected states. This is possibly due to the elevated energetic demand of osmoregulation, as well as the water loss experienced during acclimation to high salinities.
There is growing evidence that increased susceptibility to WSD is due to an inability of osmotically stressed shrimp to mount a regular immune response. These responses have been characterized by changes in hematological and biochemical variables. For example, total hemolymph [fluid, analogous to the blood in vertebrates, that circulates in insects and crustaceans] protein levels were shown to decrease in shrimp following exposure to osmotic stress combined with WSSV infection. Hemocytes are responsible for clotting and the elimination of foreign materials from the hemolymph, and reductions in levels of hemocytes are strongly correlated with increased susceptibility to pathogens. Coagulation at WSSV injection sites, phagocytosis [process to engulf and destroy microorganisms], and cell lysis [breakage of cell wall] during osmoregulation are the assumed causes of reduced THC, with the additional reduction due to salinity stress likely playing an important role in modifying the shrimp’s ability to produce a normal immune response.
Researchers have shown that L. vannamei susceptibility to WSD was constant at a range of salinities from 2 to 35 ppt, except at 15 ppt where infections in the gills and gastric epithelia were more severe. The combined effects of possible cellular damage, increased osmoregulation requirements, and reduced immune parameters are likely to explain the increased susceptibility of shrimp to WSD at non iso-osmotic salinities. This is supported by evidence that susceptibility to other shrimp pathogens – Yellow Head Virus (YHV), Taura Syndrome Virus (TSV), Vibrio alginolyticus and others – also increased in penaeids reared under those conditions.
Implications for salinity management on farms
At a range of salinities from 0 to 40 ppt, severe infection levels can occur rapidly, indicating that modifying salinity alone will not be sufficient to deter WSSV outbreaks. At salinities of 25 ppt, shrimp demonstrate a greater resilience to infection, with enhanced immune parameters and greater energy availability due to the diminished need for continuous osmoregulation and stress responses. However, the results of immune function studies must be interpreted carefully and considering the experimental design adopted, because at the time of the WSSV challenge the shrimp’s immune systems may have been fatigued following periods of acclimation to suboptimal salinities (and salinity stress).
https://www.aquaculturealliance.org/advocate/influence-of-stressors-on-shrimp-susceptibility-to-white-spot-disease-part-2/
Now that you've reached the end of the article ...
… please consider supporting GSA’s mission to advance responsible seafood practices through education, advocacy and third-party assurances. The Advocate aims to document the evolution of responsible seafood practices and share the expansive knowledge of our vast network of contributors.
By becoming a Global Seafood Alliance member, you’re ensuring that all of the pre-competitive work we do through member benefits, resources and events can continue. Individual membership costs just $50 a year.
Not a GSA member? Join us.
Authors
-
Rebecca S. Millard
Corresponding author and Ph.D. student
Biosciences, College of Life and Environmental Sciences
University of Exeter
Stocker Road, Exeter EX4 4QD, United Kingdom[107,117,46,99,97,46,114,101,116,101,120,101,64,55,50,53,109,114]
-
Robert P. Ellis, Ph.D.
Biosciences, College of Life and Environmental Sciences
University of Exeter
Stocker Road, Exeter EX4 4QD, United Kingdom -
Kelly S. Bateman, Ph.D.
Centre for Sustainable Aquaculture Futures
University of Exeter
Stocker Road, Exeter EX4 4QD, United Kingdom -
Lisa K. Bickley, Ph.D.
Biosciences, College of Life and Environmental Sciences
University of Exeter
Stocker Road, Exeter EX4 4QD, United Kingdom -
Charles R. Tyler, Ph.D.
Biosciences, College of Life and Environmental Sciences
University of Exeter
Stocker Road, Exeter EX4 4QD, United Kingdom -
Ronny van Aerle, Ph.D.
Centre for Sustainable Aquaculture Futures
University of Exeter
Stocker Road, Exeter EX4 4QD, United Kingdom -
Eduarda M. Santos, Ph.D.
Biosciences, College of Life and Environmental Sciences
University of Exeter
Stocker Road, Exeter EX4 4QD, United Kingdom
Tagged With
Related Posts
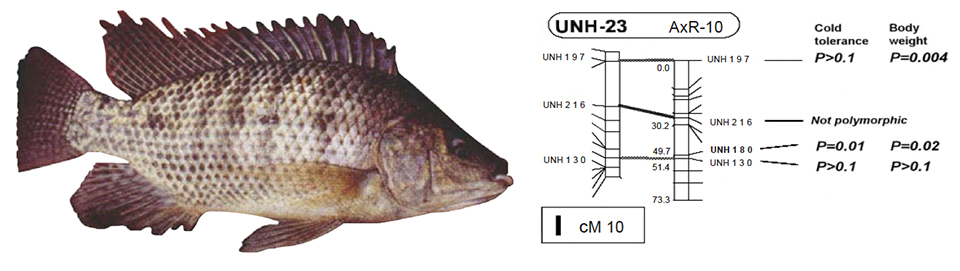
Health & Welfare
A look at aquaculture genomics
Advances in genomics assist aquaculture science by deepening the understanding of adaptation, physiology and quantitative genetics.
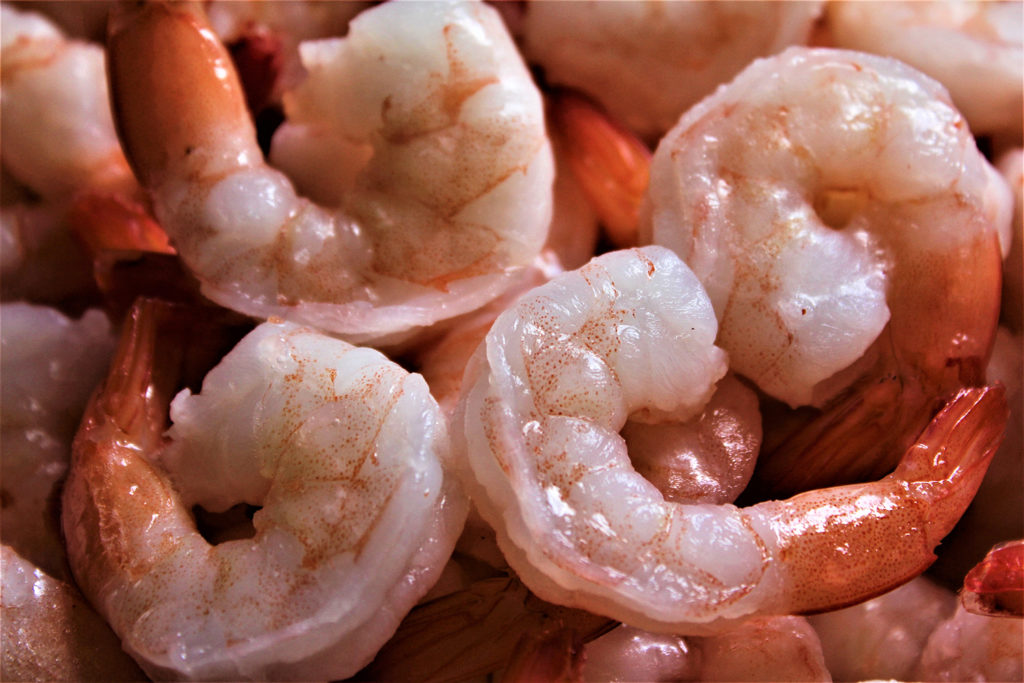
Health & Welfare
Assessment of transmission risk in cooked, WSSV-infected shrimp
Exported cooked shrimp infected with White Spot Syndrome Virus (WSSV) and tested positive by PCR is considered a risk factor for the introduction of the pathogen.
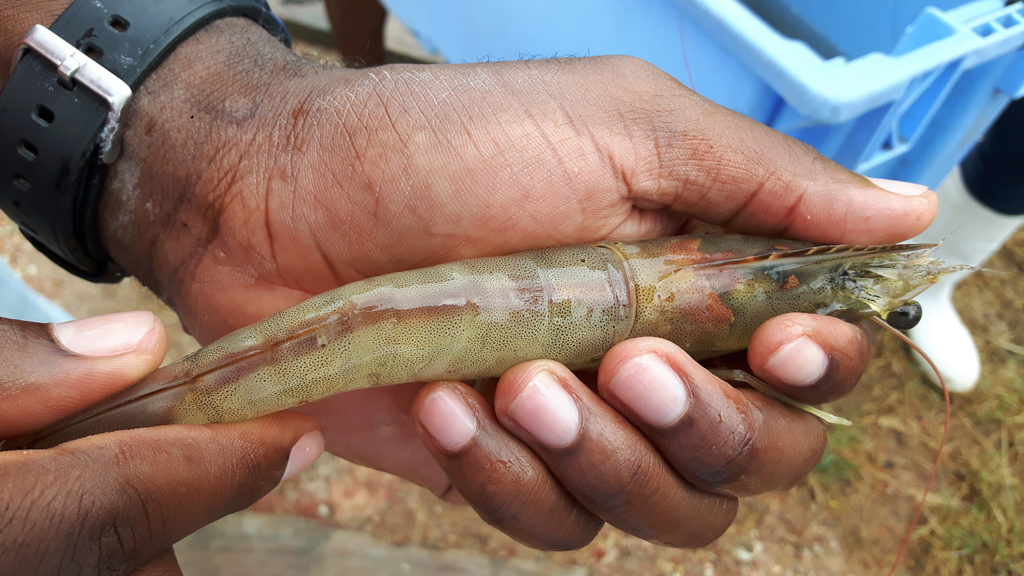
Health & Welfare
Quality, survival of L. vannamei offspring from ablated, non-ablated females
Evaluating the quality and survival of Pacific white shrimp postlarvae from non-ablated female broodstock, a holistic biosecurity and management strategy.
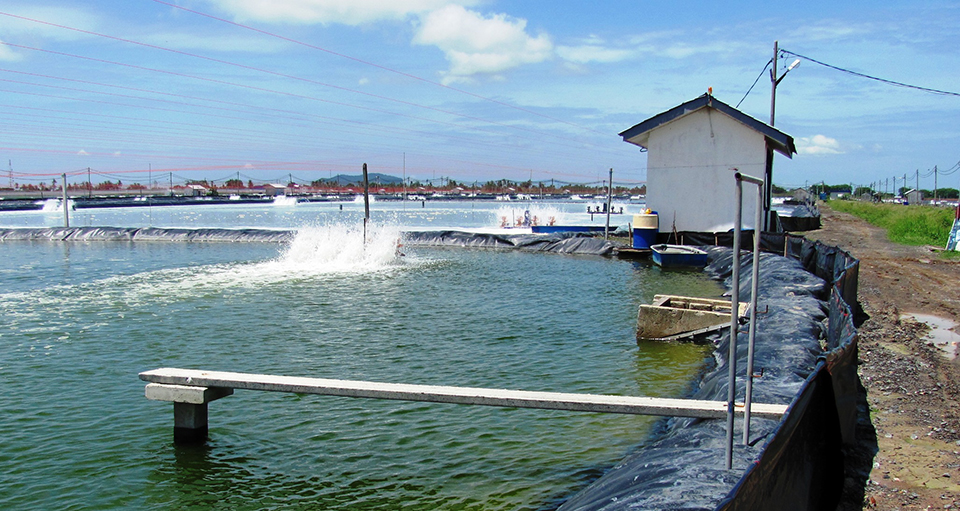
Health & Welfare
Biosecurity for shrimp farms
With the global spread of viruses, biosecurity has become an essential element of every shrimp farm. Biosecurity starts with quality of farm design.