Quantitative trait loci include temperature tolerance, spawning time and developmental rate

Genomics – the study of genome structure and function – is a rapidly developing field at the interface of quantitative and molecular genetics. A growing body of genomics research focuses on aquaculture species and production-related traits.
One well-established genomic research approach is to screen molecular genetic markers and performance traits within families to detect genes that influence expression of the performance traits. The ultimate goal is to utilize the findings for genetic marker-assisted selection for improvement of the traits.
In a newer approach, DNA sequences are spotted onto a microarray and screened to identify genes whose expression increases or decreases in response to a given stimulus.
Quantitative trait loci
The most common application of genomics is to detect genes that affect a trait of interest, termed quantitative trait loci or QTLs. A number of QTLs have been detected in fish, including those for upper thermal tolerance, spawning time, and developmental rate in rainbow trout. QTLs affecting cold tolerance and salinity tolerance have been detected in tilapia.
In each case, quantitative trait loci were detected in backcrosses or F2 crosses of species, inbred lines, or divergently selected lines – that is, in family material not generally representative of aquaculture stocks. Can such findings be extended to outbred populations? Recent work suggests they can.
The Danzmann-Ferguson group at the University of Guelph in Canada has linked three genetic markers with upper thermal tolerance in divergently selected lines of rainbow trout. More recently, the group sought to determine whether these markers showed the same association in two outbred stocks.
The researchers crossed four males and four females of two commercial strains, and exposed the third generation to thermal challenge. Their results showed, for example, that inheritance of different marker alleles at a locus for sire 92-32-1 explained 7.5 percent of the variation for upper thermal tolerance – a QTL of large effect. A model incorporating phenotype, marker allele, and pedigree information for all four sires showed that grandparental alleles were associated with different degrees of upper thermal tolerance in grand-progeny. However, the genetic markers had a stronger association with upper thermal tolerance in divergently selected lines than outbred families.
These results exemplify how findings from family material developed for genetic-mapping purposes can be used as the basis for screening for QTLs in outbred populations. As genetic maps for cultured salmonids, tilapia, catfish, shrimp, and oysters become more elaborated, QTL detection becomes more practical.
When the genetic architecture of a trait becomes known, marker-assisted selection becomes a practical approach for genetic improvement. The genetically marked, performance-increasing alleles can be selected for within the stock used for QTL detection or introgressed into another stock by appropriate crosses and marker-assisted selection.
Stress response
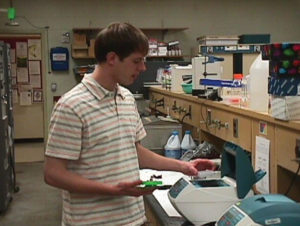
Animals are often subjected to environmentally induced stressors such as temperature, salinity, nutrient limitation, or disease. Genomics methods can help identify genes that contribute to stress resistance.
For example, Gidi Hulata and colleagues in Israel identified genetic markers linked to QTLs for cold tolerance in linkage group 23 of tilapia. Aquaculture scientists would like to go further, identifying the actual genes in key stress response pathways.
They can choose between two approaches. First, with knowledge of genetic markers linked to QTLs, they could utilize bacterial artificial chromosomes to painstakingly close in on and clone the genes of interest. Alternatively, the researchers could use microarrays, perhaps comparing gene expression on identical microarrays among individuals that were or were not stressed in a way of interest. Such work has been undertaken to characterize the responses of tilapia to Streptococcus iniae, of eastern oyster to Dermo Disease, and of shrimp to Taura Syndrome Virus.
Animals respond to environmental cues like temperature and day length to initiate key life events, such as migration, gonadal or gametic maturation, spawning, and smoltification. While scientists may know the key tissues, hormones, and receptors involved with these processes, their knowledge is incomplete. The pathways by which environmental cues are transduced into hormonal cascades are generally not known. To identify these molecular genetic pathways, microarrays might be used to identify candidate genes expressed in brain, gonad, or other key organs in response to known or suspected environmental cues.
Inbreeding depression

Breeders and aquaculturists would like to know the genes and mechanisms underlying the inbreeding depression that can decrease the performance of aquaculture stocks. Genomics-based approaches can yield new insights.
The classical view is that inbreeding depression is the result of small effects summed across many loci. However, inferring the effects of segregating alleles at individual loci has proven impossible using classical methods. An interesting case study outside of aquaculture showed how genome mapping can contribute to greater understanding.
Conifers are outcrossers that exhibit high levels of inbreeding depression. Remington et al. screened for QTLs for early survival and early growth in a selfed family of loblolly pine. Just two QTLs linked to height growth accounted for 13 percent of the inbreeding depression. One, mapped to a lignin synthesis mutation, offered an explanation for phenotypic variation. A dominant chlorophyll synthesis mutation had significant effects upon survival.
Together, the results suggested that genes affecting inbreeding depression are largely life stage-specific, and challenge the view that inbreeding depression for these traits is due to small effects across many loci. This case study showed how the genomics approach provided detail about inbreeding depression not otherwise attainable. Similar studies with aquatic organisms have been undertaken by Hedgecock and Guo, for example, to detect segregation distorter genes in eastern oysters.
An alternative way to identify the mechanisms underlying inbreeding depression might use microarrrays to identify genes that are differentially expressed in inbred and outbred half-siblings.
Molecular basis for heterosis
Some interspecific hybrids and intraspecific crossbreds express positive performance gains over parents for valued traits. The molecular basis for this heterosis is generally not understood. Is it due to the expression of novel molecules, such as the novel hemoglobin in hybrid sunfishes? This is likely the exceptional case.
Is heterosis due to novel gene regulation, perhaps mediated by simple dominance? This basis may be more common. Perhaps the expression of which genes are affected by hybridization? Genomic approaches might yield interesting insights, perhaps by a combination of QTL mapping and microarray analysis of gene expression.
Editor’s Note: Cited references are available from the author.
(Editor’s Note: This article was originally published in the September/October 2006 print edition of the Global Aquaculture Advocate.)
Now that you've reached the end of the article ...
… please consider supporting GSA’s mission to advance responsible seafood practices through education, advocacy and third-party assurances. The Advocate aims to document the evolution of responsible seafood practices and share the expansive knowledge of our vast network of contributors.
By becoming a Global Seafood Alliance member, you’re ensuring that all of the pre-competitive work we do through member benefits, resources and events can continue. Individual membership costs just $50 a year.
Not a GSA member? Join us.
Author
-
Eric M. Hallerman, Ph.D.
Department of Fisheries and Wildlife Sciences
Virginia Polytechnic Institute and State University
Blacksburg, Virginia 24061 USA[117,100,101,46,116,118,64,109,114,101,108,108,97,104,101]
Tagged With
Related Posts
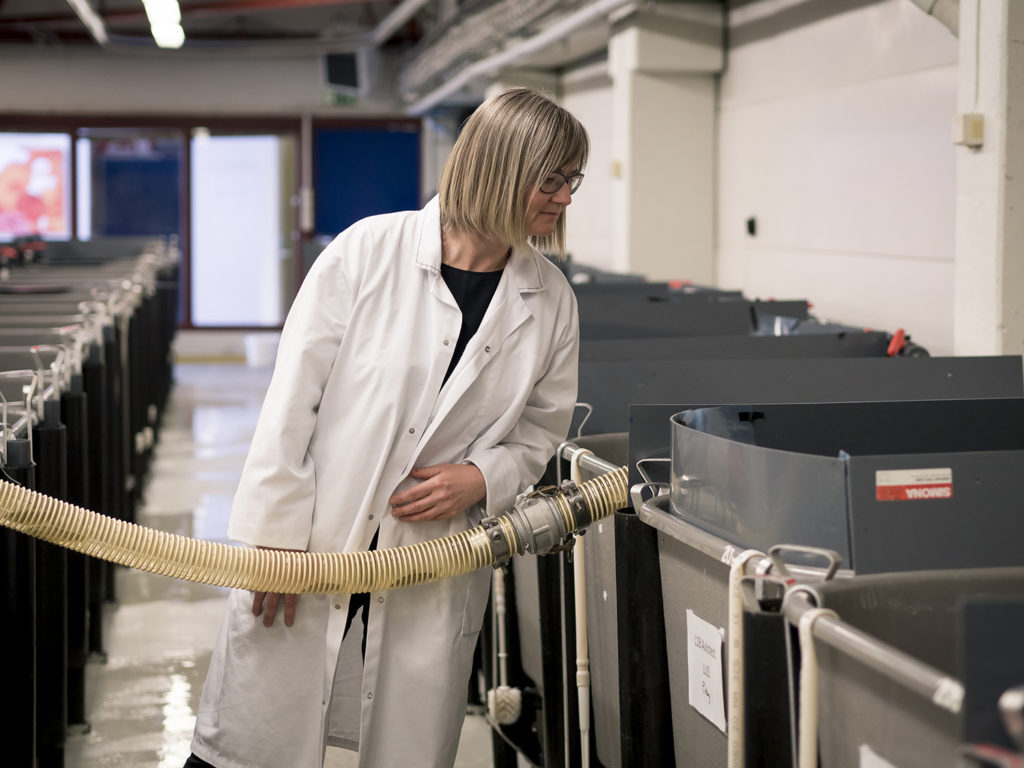
Innovation & Investment
AquaGen CEO: Genomics are transforming aquaculture
The CEO of AquaGen knew that the Norwegian research group’s work in genomics was key to the salmon industry’s future. And that was before she even worked there.
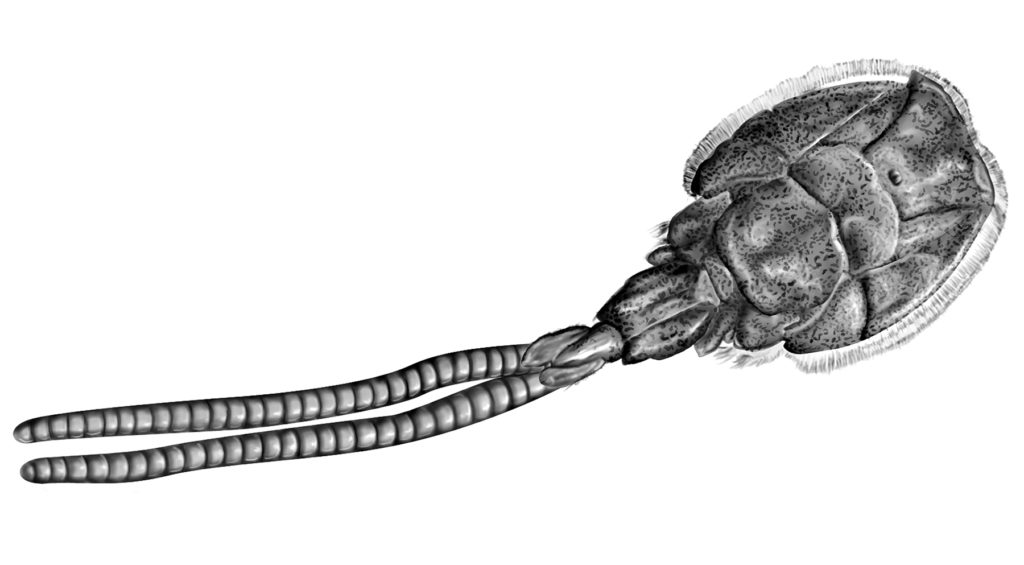
Health & Welfare
Animal health giants have sea lice in their crosshairs
Alltech and Benchmark have been working on the next generation of sea lice solutions and believe they have new products that can help salmon farmers win.
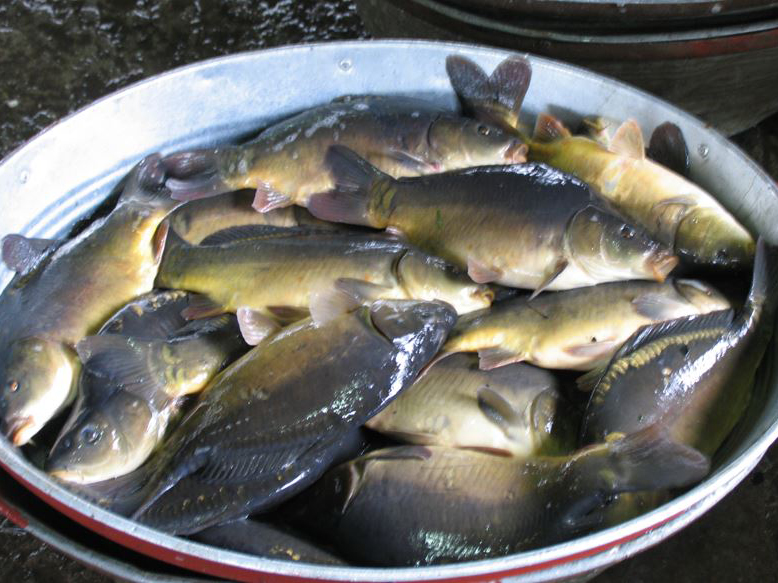
Health & Welfare
Barcoding, nucleic acid sequencing are powerful resources for aquaculture
DNA barcoding and nucleic acid sequencing technologies are important tools to build and maintain an identification library of aquacultured and other aquatic species that is accessible online for the scientific, commercial and regulatory communities.
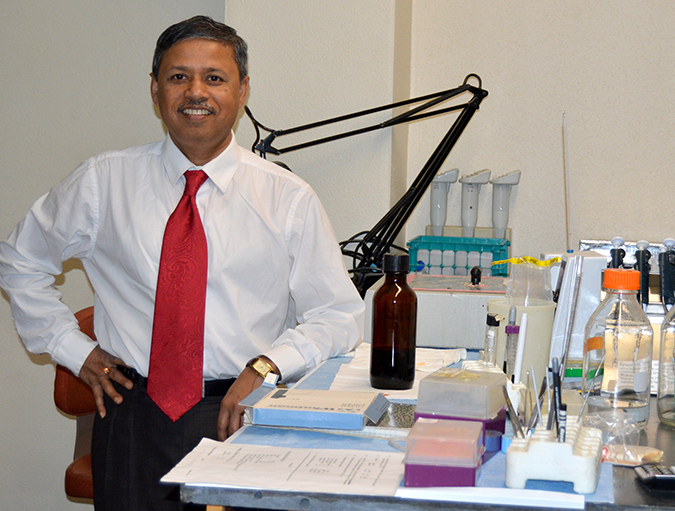
Health & Welfare
Big shoes to fill: Dhar takes reins at shrimp pathology laboratory
Arun Dhar, Ph.D. will attempt to fill the “big shoes” of Dr. Donald Lightner at the University of Arizona’s Aquaculture Pathology Laboratory, where the shrimp disease EMS was diagnosed.