Expediting genetic gains, technical challenges, public and regulatory acceptance, perspectives

Editor’s note: this article is part 2 of the original publication, adapted and summarized here. Part 1 can be found here.
Fixing alleles at existing QTL
Detecting and utilizing causative variants for a quantitative trait locus [QTL; a locus (section of DNA) that correlates with variation of a quantitative trait in the phenotype of a population of organisms]; often an early step in identifying and sequencing the actual genes that cause the trait variation. Effecting production traits is a fundamental goal of most animal breeding and genetics research, albeit with few success stories to date. Simulations have demonstrated that harnessing genome editing for favorable causative alleles at multiple QTLs as part of a breeding program, has the potential to expedite genetic gain compared with pedigree or genomic selection alone.
However, a major challenge for the effective application of this approach is the successful identification of causative variation underpinning QTLs, particularly those of small effect. To achieve this, a suite of genetic and genomic technologies can be applied to shortlist of candidate variants identified from large-scale genome-wide association studies (Fig. 1). The same approach could be used to remove deleterious variants that are inevitable in populations, both large-effect variants (e.g., recessive lethal mutations), and more polygenic deleterious loads. However, a challenge for polygenic traits (a trait whose phenotype is influenced by more than one gene) is the need to edit multiple alleles simultaneously in the same broodstock animal(s) to achieve notable impact using this approach and, therefore, development and improvement of multiplex genome-editing (where multiple genes are manipulated) approaches is required.

A pipeline for the discovery of causative variants for complex traits of relevance to aquaculture production
Shortlisting alleged functional variants underlying QTL as potential targets for in vivo CRISPR/Cas9 will require considerable research effort, and may include: (i) gene expression comparison of animals with disparate phenotypes for the traits of interest; (ii) functional annotation of polymorphisms (the occurrence of two or more clearly different morphs or forms, also called alternative phenotypes, in the population of a species) within genomic regions of interest; (iii) comparative genomics, including phylogenetic (evolutionary) conservation of the region; and (iv) detection of variants impacting gene expression of candidate loci within these regions (e.g., expression QTL or allele-specific expression).
The impact of targeted edits in candidate genes can be tested in vivo and in vitro using a combination of gene knockout and ultimately “allele-swap” experiments using homology directed repair (HDR; a mechanism in cells to repair double-strand DNA lesions) as a template to change the unfavorable version of an allele at a QTL to a favorable version before assessing the impact on the target phenotype. This pipeline of technologies can shortlist the many thousands of candidate variants within QTL regions down to the likely causative variant. Rapid developments in genomics technologies in fish will greatly assist this process. For example, reference genome assemblies are now available for most aquaculture species, and the focus now is on improving these assemblies and in particular on functional annotation to discover functionally relevant regions (e.g., open chromatin, promoters, enhancers, etc.).
Introgression-by-editing: accessing alleles from different strains or species
One of the exciting possibilities of genome editing is to access genetic variation outside closed breeding populations, without the need for costly and time-consuming introgression [the movement of a gene (gene flow) from one species into the gene pool of another species through the repeated backcrossing of an interspecific hybrid with one of its parent species] programs, or in cases where introgression is impossible.
It is common that a particular farm animal strain, or a closely related species, has a desirable characteristic. If the alleles responsible for that intra- or interspecific variation in phenotype can be identified, then CRISPR technology potentially allows editing of the unfavorable allele in the target strain and/or species to correspond to the sequence of the favorable allele found in the related strain or species (i.e., introgression-by-editing). In other words, it offers new opportunities to bypass traditional introgression, thereby avoiding the downsides associated with linkage drag (e.g., negative effects on growth rate associated with introgressing alleles from wild strains), and it allows access to genetic variation in other strains and species that would not be possible using conventional selective-breeding methods.
From a pragmatic standpoint, the early applications of such introgression-by-editing approaches will need to hold promise for transformative impacts on production to justify the extensive research and development effort required. In Atlantic salmon, parasitic copepod sea lice (Lepeophtheirus salmonis in the Northern hemisphere and Caligus rogercresseyi in the Southern hemisphere) have a crippling impact on sustainable aquaculture, with an economic impact of more than $880 million per annum globally. A unique aspect of aquaculture is the proximity of farmed species to extant wild species and populations that may have desirable characteristics. For example, certain Pacific salmon species, such as coho salmon and pink salmon, are largely resistant to sea lice and are able to mount a successful immune response against the parasite. This raises the enticing possibility of transferring resistance mechanisms to Atlantic salmon, and substantial research efforts have been made to identify the factors underlying the relative differences in host resistance mechanisms.
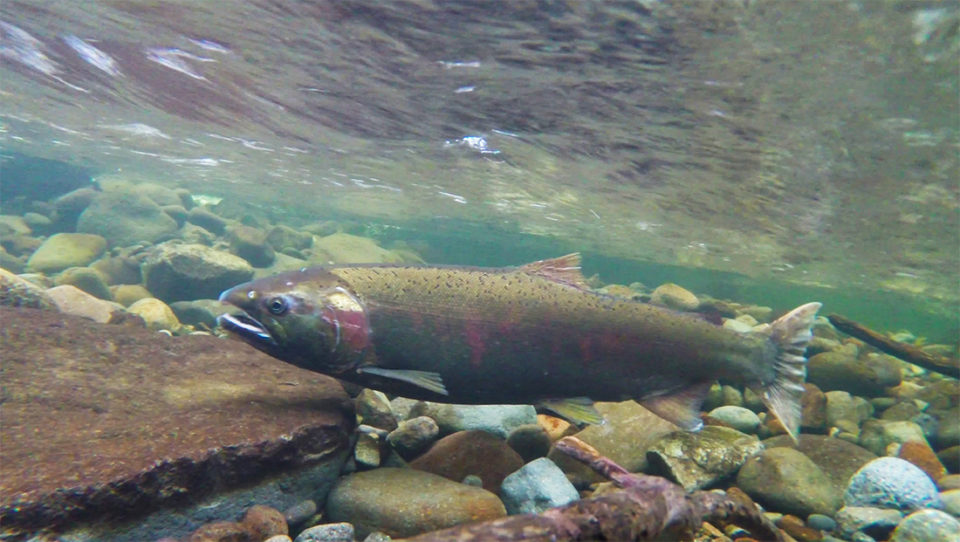
It is plausible that there are key regulatory genes in the pathways underpinning differential resistance between the species that could be modified in Atlantic salmon to mimic the response to sea lice exhibited by the coho salmon. This may be targeted editing of the coding sequence and/or modulation of the regulatory sequence to enhance or suppress the expression of these key host response genes. However, temporal and/or spatial differences in gene expression often have a significant impact on a given trait, and overall modification of expression may not achieve the desired effect.
Creating de novo variants based on knowledge of the trait
While genome editing based on existing genetic variation (either within the farmed strain, or via introgression-by-editing) gives rise to possibilities for major benefits in animal production, creating de novo favorable alleles (i.e., those that are distinct from any naturally occurring alleles, to the best of our knowledge) is another exciting avenue and has already resulted in potential solutions to animal production and welfare problems. In this approach, novel alleles can be created using CRISPR/Cas9 based on a priori knowledge of the biology of the trait of interest, or from genome-wide genetic perturbation approaches to identify candidate genes influencing the trait. An example of the former is the development of Porcine Reproductive and Respiratory Syndrome Virus (PRRSV) resistance in pigs, where genome editing was used to knockout the CD163 gene, resulting in a viable animal missing the entire receptor, or created a modified receptor by removing a specific exon and its associated protein domain.
Similar approaches have been used in aquaculture, including modification of an allele to induce sterility in Atlantic salmon and targeting the mstn1 gene in several fish species to increase growth. Alternatively, reverse genetic screens can facilitate the discovery of de novo alleles impacting traits of interest. Such genome-wide CRISPR/Cas9 screens can be performed in cell lines, and may provide de novo targets for downstream testing and potential editing in vivo, in particular for disease resistance traits.
Pooled CRISPR screens for disease resistance
One of the most powerful techniques that emerged from the advent of CRISPR/Cas9 editing is the genome-wide CRISPR knockout (GeCKO) approach. This comprises creating a library of tens of thousands of target guide RNAs (gRNA; one of two components of engineered CRISPR systems) to target every gene in the target organism, followed by synthesis, packaging into a lentivirus vector, and transduction of the cell line expressing Cas9 with a low dose aiming at approximately one gRNA integration per cell. The cell line is then screened (e.g., using a pathogen challenge) and the selected cells (surviving, fluorescently labelled, or another marker of selection) sequenced. Following selection, the enrichment or depletion of gRNAs informs the role of the target genes in the phenotype under investigation.
A major bottleneck for aquaculture research is the lack of suitable, well-tested, and characterized cell lines for many species of interest. Indeed, for many crustacean and molluscan species, there are no well-established immortalized cell lines. Developing such platforms will make genome-wide screening approaches a more realistic possibility in major aquaculture species. At present, CRISPR/Cas9 in cell culture is at a formative stage in fish species, albeit with promising early results.
Various aspects of in vitro genome editing need to be optimized in aquaculture species, including methods for the genomic integration of large inserts and optimization of which promoter to use to drive the expression of the gRNA in the different species and systems. Viral infections (and resistance to those infections) are high-priority target traits for in vitro studies using CRISPR/Cas9, because the innate mechanisms of host response are usually cell-intrinsic and, therefore, amenable to interrogation in existing immortalized cell lines.
Developing this technology would help facilitate an integration of large-scale genetic screens to inform the biology underpinning disease resistance and provide a pipeline of candidate alleles for application to commercial aquaculture breeding. The generation of Cas9 stable target animals may facilitate primary cell lines that are amenable to editing, and future development of immortalized cell lines (a population of cells from a multicellular organism which would normally not proliferate indefinitely, but because of mutation have evaded normal cellular senescence and instead can keep undergoing division. The cells can therefore be grown for prolonged periods in vitro) from target tissues and/or cell types that broaden the applicability of GeCKO approaches to a wider array of aquaculture species.
Technical challenges to overcome
There are several important technical hurdles to be addressed to maximize the possibilities to apply genome-editing approaches in aquaculture species. First, in species where CRISPR/Cas9 has already been applied, optimization of methods is required to maximize editing efficiency, minimize off-target effects, and reduce the problem of mosaicism (the presence of two or more populations of cells with different genotypes in one individual who has developed from a single fertilized egg) in the F0 generation. Off-target editing, which can result in nonspecific and unintended modification to the genome, may also lead to unintended impacts on the organism. Improved knowledge of the genome sequences of aquaculture species will assist with the design of gRNAs specific to a single targeted region, and the relatively modest cost of whole-genome resequencing can facilitate routine screening for off-target editing events.
To rapidly select optimal gRNAs, constructs can be tested in cell culture before in vivo editing. Particularly for species where access to newly fertilized embryos is difficult, such as certain shrimp species, alternative methods of delivery of CRISPR/Cas9 could be tested, including sperm-mediated transfer, microinjection of unfertilized ova, and editing primordial germ cells. Another approach to enrich for edited alleles of interest is germ cell transplantation from animals with a desired edit, into multiple sterile surrogates. Analogous approaches are being successfully used for editing in chickens, and transfer of skills and technologies from terrestrial livestock and model organisms to aquaculture species will be key to the success of this approach.
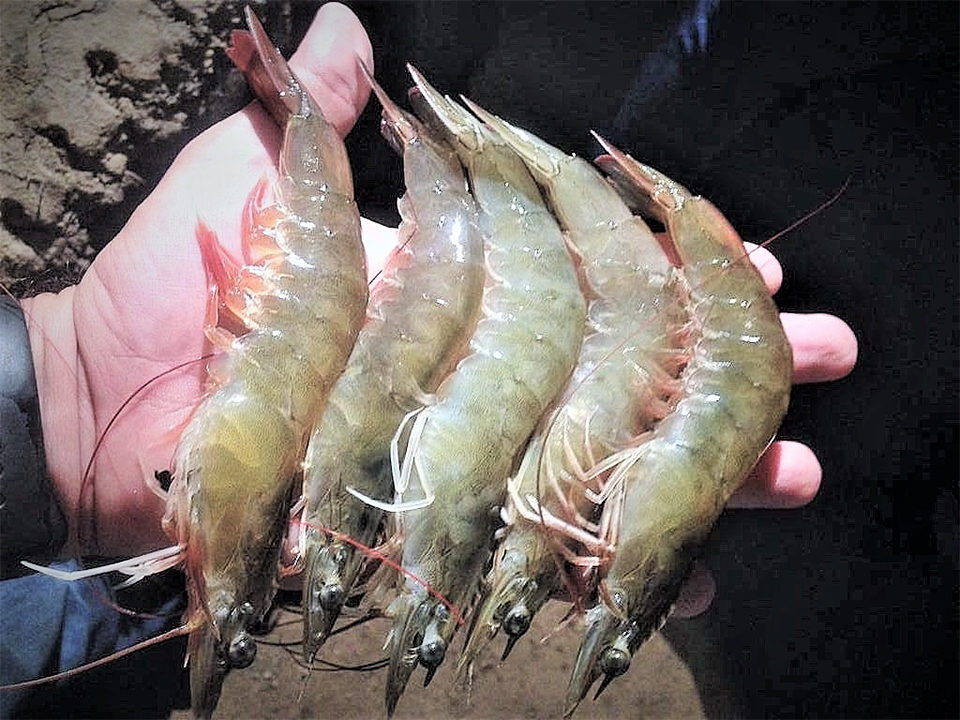
Factors affecting public and regulatory acceptance
Innovation in technology is essential to advance food production to address the increasing global demand. CRISPR/Cas9 technology has the exciting potential to contribute to the improved quantity, quality and sustainability of seafood production globally. However, public and regulatory acceptance are key to its potential being realized.
There is considerable debate about the definition of genetic modification (GM) and whether genome-editing approaches should be considered separately. If genome editing is considered separately, the different applications discussed earlier may be subject to different regulations. For example, genome editing animals could be created with just single base changes in their genome that correspond to existing polymorphisms within farmed and/or wild stocks. Alternatively, de novo alleles can be created that are absent in nature to the best of our knowledge. The former may be more acceptable to the public and could feasibly be subjected to less stringent regulatory procedures. However, the ruling by the European Court of Justice that genome edited crops should be considered GM organisms is likely to hinder the commercial-scale application of genome editing in EU-farmed species.
However, it is noteworthy that a GM salmon (the AquaBounty salmon with a transgenic growth hormone gene) has been approved for human consumption by the FDA and the Canadian Food Inspection Agency. Furthermore, a line of tilapia derived from genome editing by the same company has been exempted from GM regulation in Argentina. It is clear there will be longstanding uncertainty about the regulation of edited animals, and the process will vary considerably in different countries.
Therefore, extensive engagement with the public and other stakeholders to facilitate knowledge-driven decisions about benefits and risks of the technologies is key. From a public acceptance standpoint, it is important to consider the nature of the target traits, and whether the potential benefits stretch beyond sustainable production and profit. For example, traits such as sterility also have downstream benefits for the environment and wild stocks, and traits such as disease resistance have substantial concurrent benefits for animal welfare.
Perspectives
Aquaculture is the fastest growing food production sector, is rapidly assuming greater importance than capture fisheries, and is seen as an essential component of food and nutrition security, particularly in the developing world. The use of genetics and breeding technologies in aquaculture is rapidly increasing, with the development of high-tech breeding programs for many of the world’s most important aquaculture species. Most farmed aquatic species are close to wild ancestors, and this offers a major untapped resource to enhance sustainable seafood production from aquaculture. Furthermore, the external fertilization and high fecundity of aquaculture species offer exciting opportunities for high-resolution genetics studies to understand and improve complex traits.
Genome-editing technologies, such as CRISPR/Cas9, have significant potential to expedite genetic gain for production traits. Infectious disease is one of the primary constraints to aquaculture production and, therefore, a major target for selective breeding and genome-editing approaches. Host resistance to certain pathogens is a suitable trait for the use of genome- editing technologies due to the difficulty in nondestructive measurement of the trait in breeding candidates, the plausibility of utilizing cell culture genome-wide pooled CRISPR screens, and the frequent availability of early life in vivo-established challenge models.
Different categories of genome-editing applications include: (i) discovery of causative variants underlying single or multiple QTLs affecting traits of interest, and subsequent fixation of the favorable alleles using editing; (ii) introgression-by-editing of favorable alleles into closed breeding systems from other populations, strains, or species; and (iii) creation and use of de novo alleles with positive effects on the trait of interest. Genome editing together with established genetic and genomic approaches enables detection and shortlisting of candidate functional variants for downstream in vivo validation, and potential commercial application.
While several outstanding research priorities require major effort, the high reproductive output of most aquaculture species would enable potentially favorable alleles introduced into the germplasm of a well-managed breeding program to be disseminated at a scale and pace not feasible in terrestrial farmed animal production. Thus, subject to favorable regulatory and public perceptions, genome-editing technology has the potential to significantly transform the sustainable production of seafood through aquaculture.
Now that you've reached the end of the article ...
… please consider supporting GSA’s mission to advance responsible seafood practices through education, advocacy and third-party assurances. The Advocate aims to document the evolution of responsible seafood practices and share the expansive knowledge of our vast network of contributors.
By becoming a Global Seafood Alliance member, you’re ensuring that all of the pre-competitive work we do through member benefits, resources and events can continue. Individual membership costs just $50 a year.
Not a GSA member? Join us.
Authors
-
Remi L. Gratacap, Ph.D.
The Roslin Institute, University of Edinburgh
Easter Bush Campus
Midlothian, EH25 9RG, UK -
Anna Wargelius, Ph.D.
Institute of Marine Research
P.O. Box 1870
Nordnes, NO-5817 Bergen, Norway -
Rolf Brudvik Edvardsen, Ph.D.
Institute of Marine Research
P.O. Box 1870
Nordnes, NO-5817 Bergen, Norway -
Prof. Ross D. Houston, Ph.D.
Corresponding author
The Roslin Institute, University of Edinburgh
Easter Bush Campus, Midlothian, EH25 9RG, UK[107,117,46,99,97,46,100,101,46,110,105,108,115,111,114,64,110,111,116,115,117,111,104,46,115,115,111,114]
Tagged With
Related Posts
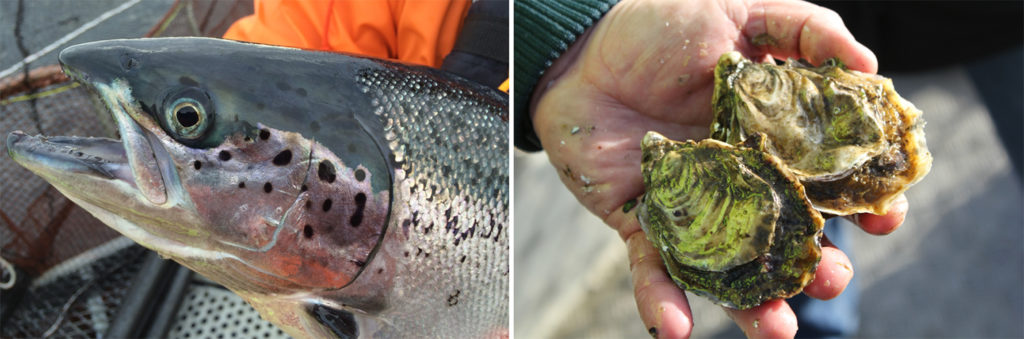
Health & Welfare
Genome editing: Potential to improve aquaculture breeding, production, Part 1
Due to high fecundity and external fertilization, most aquaculture species are amenable to genetic improvement technologies, including genome editing.
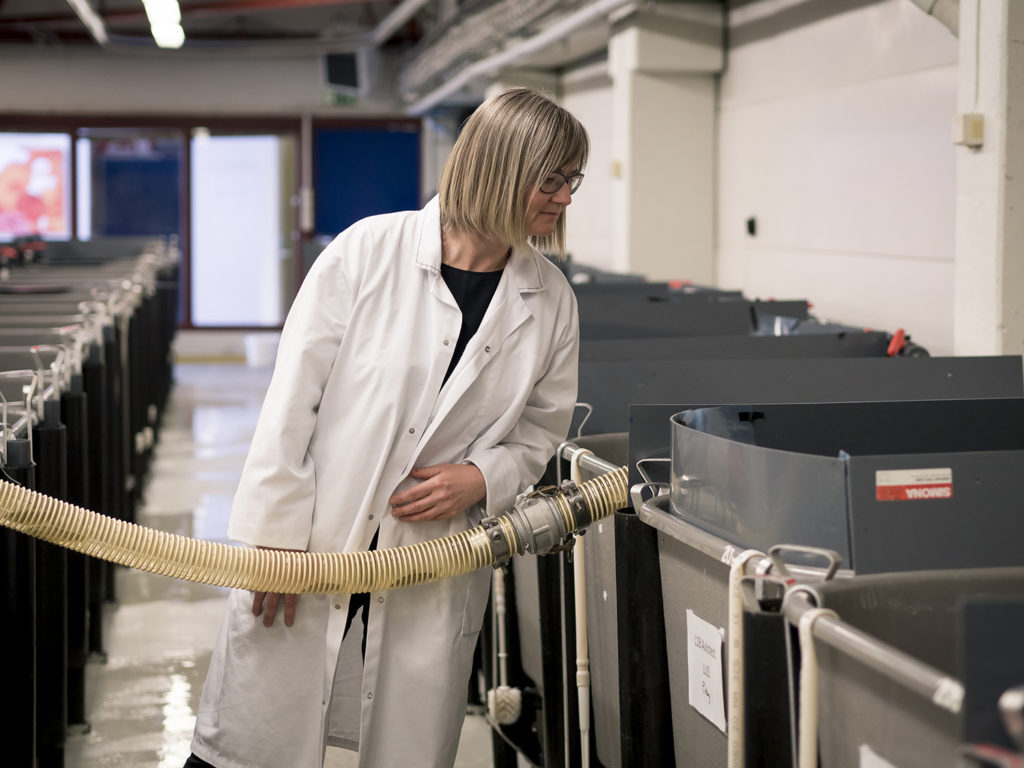
Innovation & Investment
AquaGen CEO: Genomics are transforming aquaculture
The CEO of AquaGen knew that the Norwegian research group’s work in genomics was key to the salmon industry’s future. And that was before she even worked there.
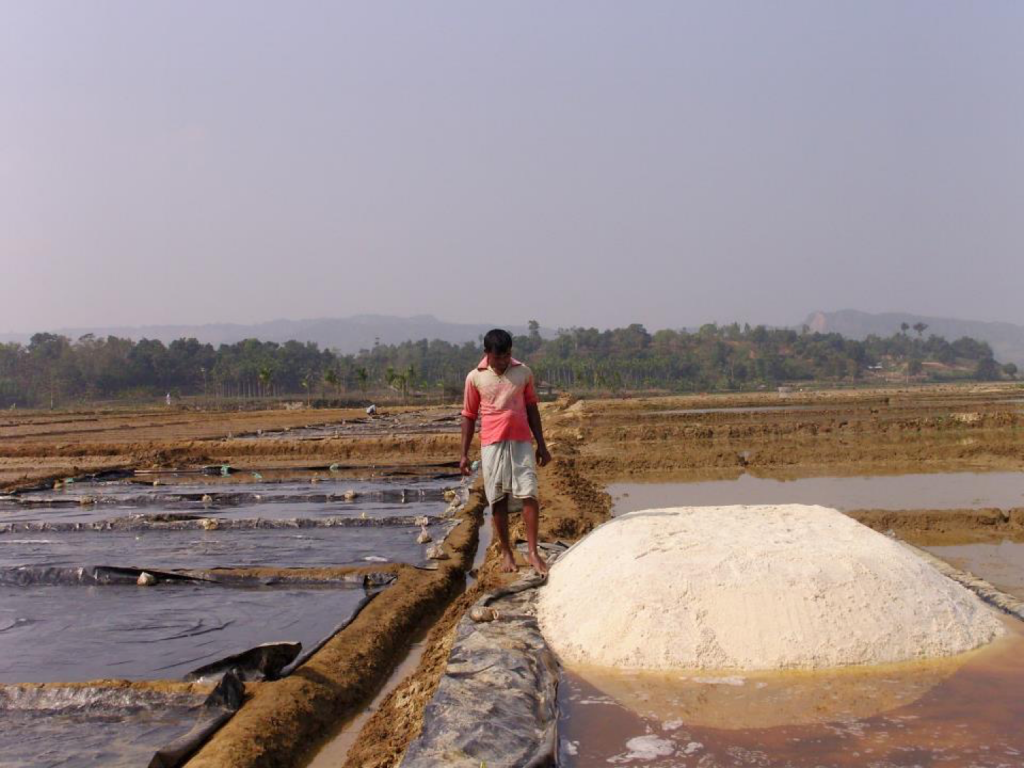
Innovation & Investment
Artemia, the ‘magic powder’ fueling a multi-billion-dollar industry
Artemia, microscopic brine shrimp used as feed in hatcheries, are the unsung heroes of aquaculture. Experts say artemia is still inspiring innovation more than 50 years after initial commercialization. These creatures are much more than Sea-Monkeys.
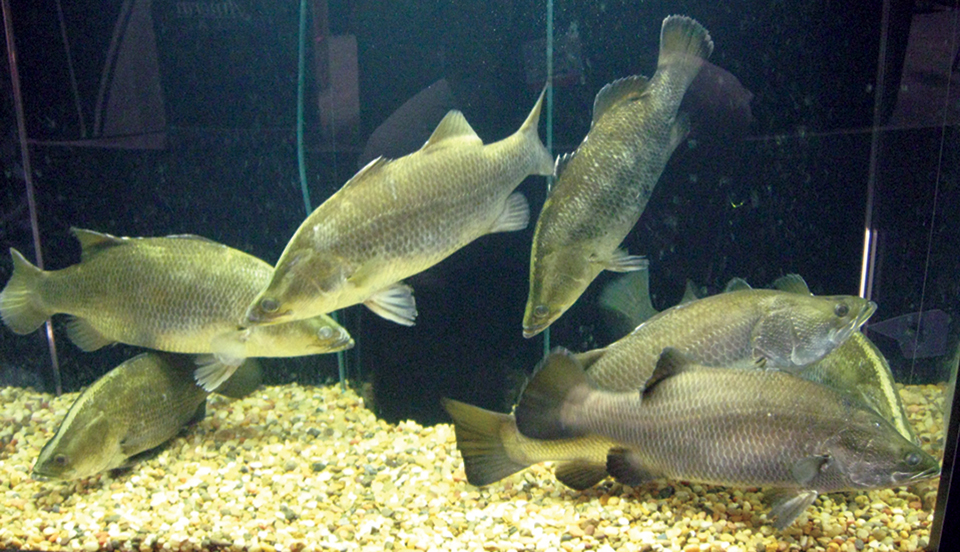
Intelligence
DNA barcoding applications in aquaculture
DNA barcoding is an exciting tool for the identification of fish. Even non-taxonomists can classify organisms through the molecular taxonomy technique.