Origin, rates, management all important considerations for aquafarmers
Before discussing organic matter decomposition, it will be helpful to mention organic matter production by plants. The end product of photosynthesis is simple sugar (C6H12O6). Plants use some of this sugar directly for energy or convert it to starch for a reserve energy source. They also use the sugar from photosynthesis to make proteins, hemicellulose, cellulose, lignin, fats, waxes, etc. that comprise plant biomass. The composition of plant parts differ and the average composition of entire plants vary among species.
Plants and organic matter
Plants are harvested and used for human and animal food and for other purposes. Plants harvested for human use are processed with resulting wastes and plants die and enter the environment as dead organic matter. The composition of organic matter is chemically complex, and livestock manures have been greatly altered from their original form by passing through the gastrointestinal tracts of animals.
Organic matter added to aquaculture production systems is some combination of organic fertilizer, uneaten feed, feces and remains of plankton and other dead organisms. Decomposition of organic matter exerts an oxygen demand and too much organic matter may lead to dissolved oxygen depletion, which is of concern in feed-based aquaculture. In fertilized ponds, organic matter is a source of food for benthic organisms that can also be eaten directly by some fish and shrimp.
Organic fertilizer for aquaculture is mainly of plant origin (Table 1). Aquaculture feeds contain mostly plant meals, but some also contain a large amount of fish meal or other animal byproduct meals. Phytoplankton make up most of the organic matter produced in ponds and other outdoor, static waters.
Boyd, decomposition, Table 1
Organic material | Concentration (% dry weight) Carbon | Concentration (% dry weight) Nitrogen |
---|---|---|
Livestock manure: Swine | 40.6 | 3.01 |
Livestock manure: Dairy | 38.2 | 2.98 |
Livestock manure: Cattle | 43.0 | 2.92 |
Livestock manure: Chicken | 32.5 | 4.47 |
Grasses | 38 to 42 | 1.5 to 3.0 |
Crop wastes | 35 to 40 | 0.75 to 2.0 |
Feed (uneaten) | 38 to 42 | 4 to 7 |
Fish manure | (less than uneaten feed) | (less than uneaten feed) |
Phytoplankton | 45 to 50 | 8 to 12 |
The carbon and nitrogen concentrations of organic materials in aquaculture are presented on a dry weight basis in Table 1. Although nitrogen concentration varies greatly among materials, carbon concentration is less variable and ranges from an average of 32.5 percent in chicken manure to 50 percent in phytoplankton. Usually, foliage used as organic matter contains 45 to 50 percent carbon.
The total amount of oxygen used by bacteria to completely oxidize carbon in 1 kg or organic materials is 2.67 times the decimal fraction of carbon, because C + O2 = CO2 and the molecular weight of oxygen is 2.67 times greater than that of carbon. For example, oxidation of 1 kg dry weight of uneaten feed containing 38 percent organic carbon would require 1.01 kg O2 [(0.38 kg C/kg feed) × 2.67 kg O2/kg organic C].
Decomposition rates
Although the above is true, the actual situation is more complex, because most organic matter does not completely decompose in a short period, and rates that different types of organic matter decompose initially vary because of differences in composition. The complete decomposition of organic materials shown in Table 1 would require many years. Of course, sugar and molasses used in biofloc aquaculture decompose very quickly and almost completely.
Sugar, starch, cellulose and protein that decompose quickly are called labile organic matter. Lignins, tannins, fats and waxes that decompose at a slower rate are known as recalcitrant (or refractory) organic matter. As organic matter decomposes, the recalcitrant fraction increases and bacterial remains also decompose. Organic matter from dead, decaying bacterial cells mixes with the recalcitrant fraction making the remaining organic matter even more complex.
In raceways, other flow-through systems, and water-recirculating systems, organic matter partially decomposes, but most of it is flushed out in effluent or mechanically removed. In ponds and in water bodies containing cages, organic matter settles to the bottom. It forms a flocculent layer of high suspended solids concentration of a few millimeters to a centimeter or more thick on the bottom.
Organic matter in the flocculent layer decomposes and its remains gradually are mixed into the bottom soil (same as sediment). In lakes with cage culture, organic matter accumulation may sometimes occur on the bottom. Bottom soil is stirred by action of organisms and water currents to a depth of a few centimeters.
Organic matter concentration is greatest in the upper 2 to 5 centimeters of the bottom soil. Within this layer, decomposition of organic matter is rapid and dissolved oxygen depletion occurs in the pore water. As a result, aerobic decomposition of organic matter in sediment decreases very rapidly with depth below the soil-water interface, but when dissolved oxygen is depleted, decomposition by anaerobic bacteria continues. In most aquaculture ponds, and especially in those with mechanical aeration, the soil to depths of down to about 1 cm below the soil-water interface usually remains aerobic.
Labile organic matter is decomposed aerobically within the flocculent layer and in the aerobic zone of the bottom soil. The recalcitrant fraction is mixed into the underlying soil and continues to decompose but at a slow rate. The problem with high oxygen demand in pond bottoms results primarily from fresh organic matter deposited during the grow-out period rather than from accumulation of recalcitrant organic matter from previous crops.
There is a continuous “rain” of organic particles and of mineral soil particles originating from inflow or from erosion within the pond. In ponds without sediment removal, sediment accumulation rate ranges from as much as 5 to 10 cm/year for the first 2 to 3 years while pond embankments are most susceptible to erosion to an average of 1.0 to 1.5 cm/year later. Older organic matter is continually buried under newer organic matter, and only the upper few centimeters – usually about 5 cm – influence pond water quality.
Organic matter concentration in the upper 5 cm of soil is usually in the range of 6 to 10 percent, while the soil beneath usually has 3 to 5 percent organic matter. However, the ratio of labile:recalcitrant organic matter decreases with greater soil depth in the upper layers.
Draining ponds for harvest results in resuspension and removal of much of the flocculent layer in effluent. Channel catfish and inland shrimp ponds in the United States often are operated for 10 to 15 years without complete draining or bottom dry-out. These observations suggest that aside from sanitation purposes, it is not necessary to remove soft sediment and dry pond bottoms after each crop or even annually.
Pond-bottom management
The practice of sediment removal and dry-out seems entrenched in the culture of many aquaculturists outside the United States. There is no question about sediment removal and pond dry-out lessening the amount of labile organic matter in pond bottoms before the next crop cycle. The question is whether the practice usually is beneficial aside from sanitation purposes, because fresh organic matter will create a large oxygen demand during the next grow-out period anyway.
A few additional comments about pond bottom soil organic matter are necessary. Many fish and shrimp farmers apply bacterial products that supposedly improve water quality and bottom soil condition. Research findings do not support this practice.
It is completely natural for bottom soil to be anaerobic at depths of 1 cm or less below the soil-water interface. There is no reason to measure oxidation-reduction potential (ORP) to confirm this.
Black soil or darker colored soil beneath the soil-water interface is completely normal. It occurs when soil is anaerobic and contains ferrous iron. As long as the soil surface (soil-water interface) does not become black or dark colored, nitrite and hydrogen sulfide produced in anaerobic decomposition will not enter the water column faster than they oxidize to acceptable levels.
It usually is not necessary to measure hydrogen sulfide. This measurement is not easy to make, test kits for hydrogen sulfide give only an indication of its presence, and the odorous smell of hydrogen sulfide is obvious at harmful concentrations – it has the unmistakably offensive odor of a rotten egg.
Liming pond bottoms with soil pH less than 7 favors greater organic matter decomposition. Of course, this is not necessarily a positive event as far as dissolved oxygen is concerned, but ponds with low pH soils usually have poorly buffered low-alkalinity waters and require liming.
The solubility of agricultural limestone and other liming materials is increased by higher organic matter concentrations in soil and by the application of organic fertilizer with liming material. This results because carbon dioxide from organic matter decomposition reacts with liming materials to increase the rate and extent of dissolution as shown by the following equation in which calcium carbonate is a type of liming material:
More carbon dioxide drives the reaction further to the right.
The carbon/nitrogen (C/N) ratio of organic matter has a large effect with a lower ratio favoring more rapid decomposition. The C/N ratio will be discussed in another article.
Managing dissolved oxygen
Management of ponds to maintain adequate dissolved oxygen within the water column is the backbone of effective water quality management, and it will also prevent most problems with organic matter in pond bottoms. The objective should be to maintain stocking rates, fertilizer rates and daily feed inputs with the limits imposed by dissolved oxygen concentrations. This necessitates balancing aeration rate with feed input in feed-based aquaculture.
Now that you've reached the end of the article ...
… please consider supporting GSA’s mission to advance responsible seafood practices through education, advocacy and third-party assurances. The Advocate aims to document the evolution of responsible seafood practices and share the expansive knowledge of our vast network of contributors.
By becoming a Global Seafood Alliance member, you’re ensuring that all of the pre-competitive work we do through member benefits, resources and events can continue. Individual membership costs just $50 a year.
Not a GSA member? Join us.
Author
-
Claude E. Boyd, Ph.D.
School of Fisheries, Aquaculture and Aquatic Sciences
Auburn University
Auburn, Alabama 36849 USA[117,100,101,46,110,114,117,98,117,97,64,49,101,99,100,121,111,98]
Tagged With
Related Posts
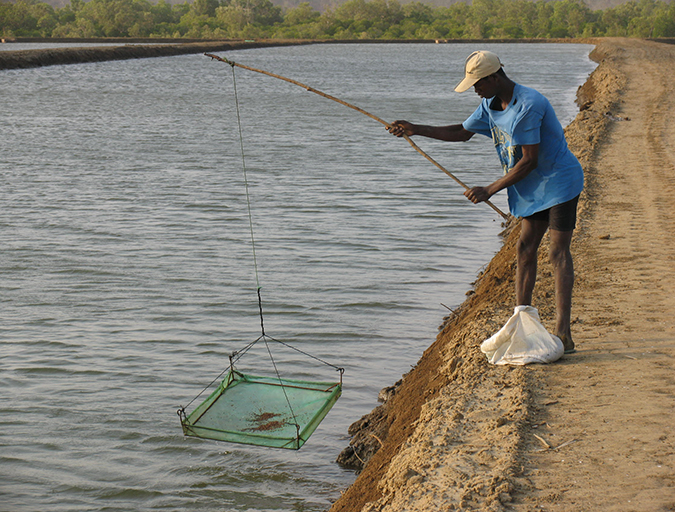
Health & Welfare
Decomposition and accumulation of organic matter in ponds
Aquaculture ponds accumulate organic matter from organic fertilizer, remains of microorganisms produced within the pond, feces of the culture animals and uneaten feed. Claude E. Boyd, Ph.D., details the leading organic matter management practices, and says that the accumulation of organic matter is often not as great as believed.
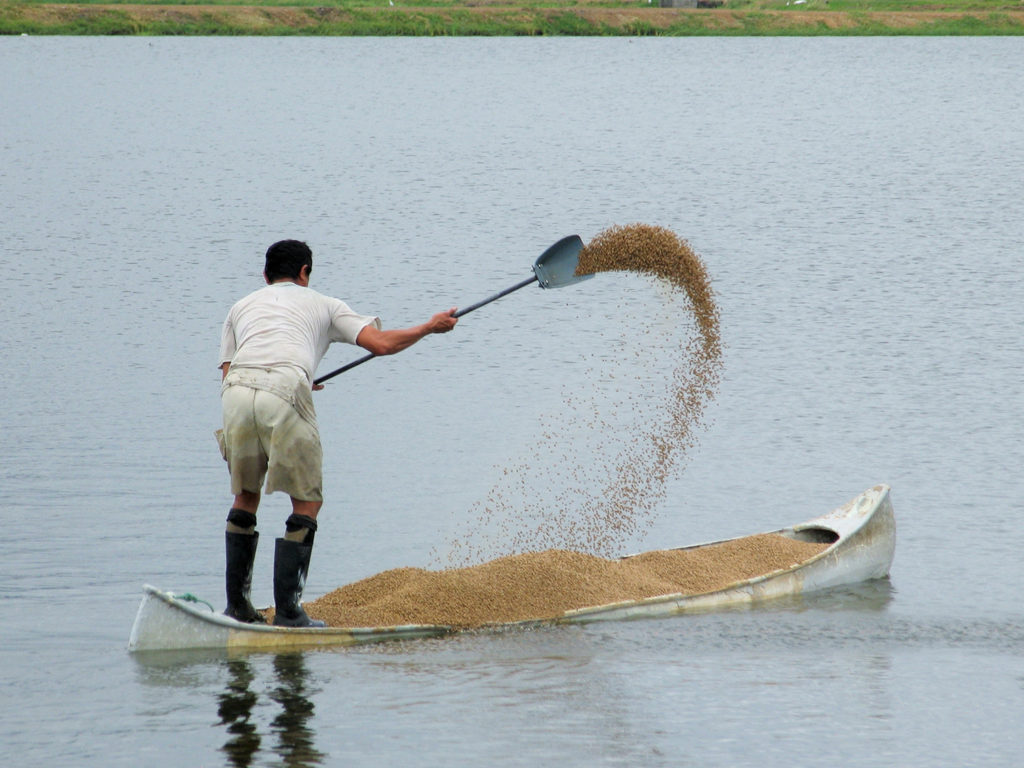
Responsibility
Ammonia nitrogen dynamics in aquaculture
The major sources of ammonia in aquaculture ponds are fertilizers and feeds, and problems with high ammonia are most common in feed-based aquaculture.
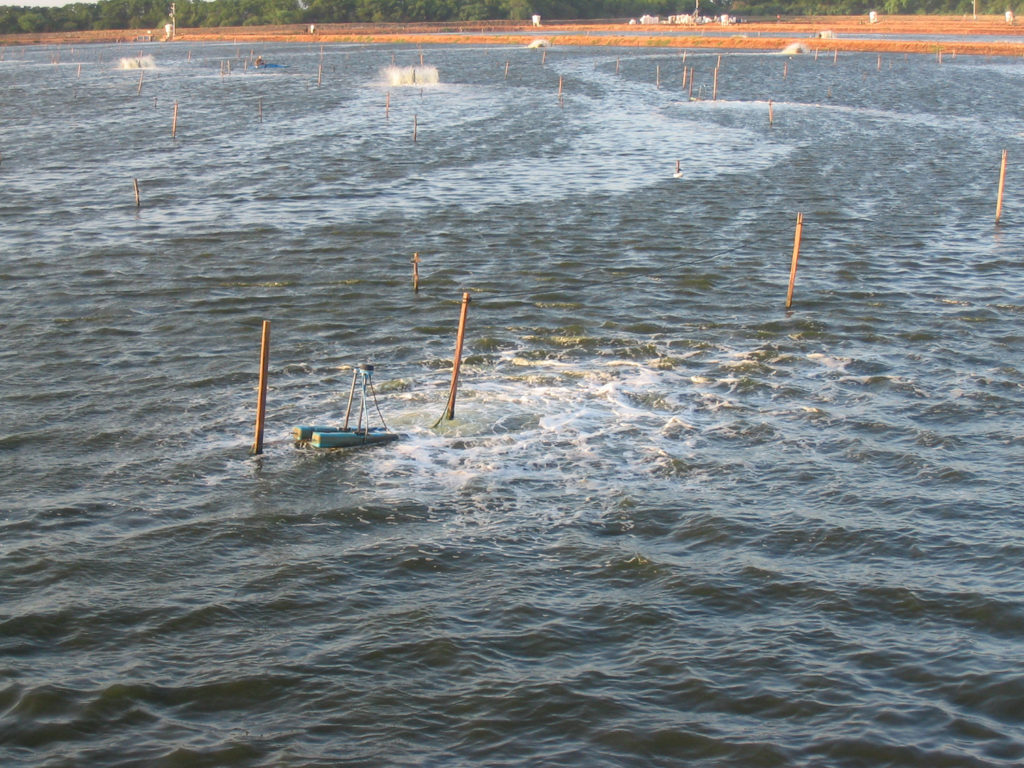
Health & Welfare
Dissolved oxygen dynamics
Dissolved oxygen management is the most important requirement of aquaculture pond water quality. DO concentration below 3 mg/L is stressful to shrimp.
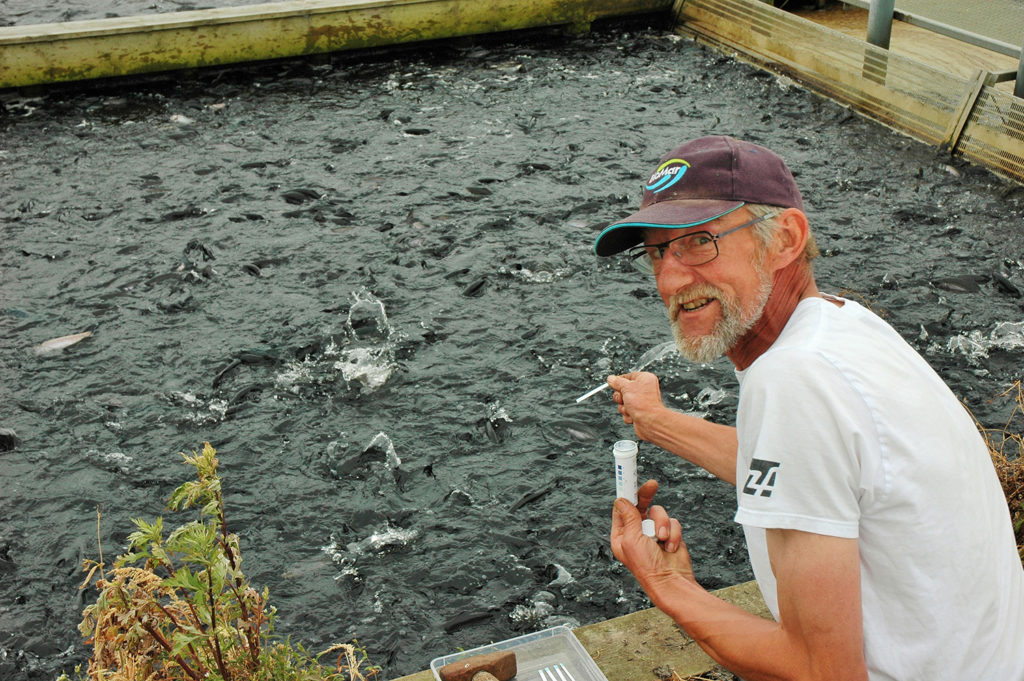
Responsibility
Microbial activity assessment based on hydrogen peroxide decomposition rates
Fast, easy, reliable method offers great potential for all aquaculture systems There is a widespread need for cultivation-free methods to quantify the viability of microbial communities in aquatic environments. This demand also exists within the aquaculture industry, where rapid and reliable methods for measuring bacterial activity and bioavailable organic matter in water are increasingly required.