Prof. Boyd: Proper management most important aspect of aquaculture pond water quality management
Phytoplankton productivity plays different roles in fish and shrimp culture. In extensive ponds, phytoplankton blooms are the base of the food web that supports production of culture animals. In semi-intensive culture, the increase in natural food derived from the phytoplankton-based food web supplements manufactured feed offered to culture animals.
In intensive ponds, culture animals obtain their nutritional requirements almost entirely from manufactured feed, but early in the culture period, fingerling fish and postlarval shrimp benefit from natural food organisms. In biofloc culture systems, the phytoplankton blooms decrease as they are partially or largely replaced by a bacterial floc that can serve as supplemental food for culture animals.
Phytoplankton including cyanobacteria (known more commonly as blue-green algae) also are important in ponds by producing dissolved oxygen. The amount of carbon transformed into organic matter through photosynthesis is chemically equivalent to the quantity of dissolved oxygen released into the water.
Dense blooms of phytoplankton are associated with low nighttime dissolved oxygen concentration, but phytoplankton are net producers of oxygen. This is evident from the existence of phytoplankton biomass itself. This biomass could not exist if phytoplankton were not net producers of oxygen, because it would be respired away by phytoplankton themselves. In aquaculture ponds, culture species, bacteria decomposing organic matter – or carrying out nitrification – and other non-photosynthetic organisms also respire.
Fertilizer increases plant nutrient availability and organic fertilizers are a nutrient input through microbial decomposition. Uneaten feed and feces also are an organic matter input, and feed consumed by culture animals is used for growth and as an energy source in fish and shrimp respiration. Culture animals excrete nitrogen, phosphorus and other inorganic plant nutrients. Aquaculture inputs increase organic matter loads to ponds directly and are sources of inorganic nutrients for phytoplankton and other aquatic plants that ultimately become dead organic matter and increase oxygen demand.
Phytoplankton productivity, dissolved oxygen production
Greater phytoplankton productivity increases dissolved oxygen production through photosynthesis, but this is not a panacea to dissolved oxygen management. Photosynthesis requires light and the intensity of photosynthetically available light decreases with water depth. Phytoplankton are restricted to the photic zone within which there is sufficient light for photosynthesis.
The catch is the thickness of the photic zone in ponds is mainly a function of phytoplankton bloom density. A denser bloom results in greater turbidity and less light penetration into the water column for photosynthesis. While more phytoplankton produce more dissolved oxygen than less phytoplankton, the depth (and volume) within which photosynthesis may occur shrinks as blooms becomes denser.
Daytime photosynthesis rate exceeds total daytime pond respiration rate, and dissolved oxygen concentration increases during the day. The opposite occurs at night when photosynthesis stops and respiration continues, causing a daily increase and decrease in dissolved oxygen concentration (Fig. 1). As phytoplankton bloom intensity increases, the difference in daytime and nighttime dissolved oxygen concentration increases. The peak of the daytime dissolved oxygen curve declines with water depth because of decreasing light for photosynthesis. Dense phytoplankton blooms greatly increase the frequency and severity of low dissolved oxygen concentration.
Molecular oxygen diffuses across the air-water interface of ponds. Water holds an equilibrium concentration of dissolved oxygen at a given atmospheric pressure, temperature, and salinity (Table 1). At less than the equilibrium concentration, greater pressure of oxygen in air forces oxygen to water. The opposite occurs when water has greater than the equilibrium dissolved oxygen concentration or is supersaturated with oxygen. Ponds lose oxygen by diffusion during most of the day, but during much of the night, they gain oxygen by diffusion. Wind action increases oxygen diffusion rate, but daily net diffusion (daytime gain – nighttime loss) is usually a small component of an aquaculture pond dissolved oxygen budget even when it is windy. Nevertheless, when dissolved oxygen concentration is low, oxygen diffusing from the air may be a lifesaver for culture animals.
Boyd, dissolved oxygen, Table 1
Temperature (degrees-C) | Salinity 0 g/L | Salinity 10 g/L | Salinity 20 g/L | Salinity 30 g/L | Salinity 40 g/L |
---|---|---|---|---|---|
10 | 11.28 | 10.58 | 9.93 | 9.32 | 8.75 |
15 | 10.07 | 9.47 | 8.91 | 8.38 | 7.88 |
20 | 9.08 | 8.56 | 8.07 | 7.60 | 7.17 |
25 | 8.24 | 7.79 | 7.36 | 6.95 | 6.57 |
30 | 7.54 | 7.14 | 6.76 | 6.39 | 6.05 |
35 | 6.94 | 6.58 | 6.24 | 5.92 | 5.61 |
The compensation depth is the depth at which total daily production of oxygen by photosynthesis exactly equals daily use of dissolved oxygen by respiration of microorganisms living in the water column – fish, shrimp, and other larger organisms excluded (Fig. 2). The amount by which the area of the shaded region above the compensation depth in Fig. 2 exceeds the area of the shaded region below it is the quantity of dissolved oxygen available to culture species and for use by pond bottom organisms. The more the excess of dissolved oxygen above the compensation depth exceeds the deficit of dissolved oxygen below it (Fig. 2), the less likely will be low dissolved oxygen concentration, and especially at night.
Aeration
Mechanical aeration in ponds can prevent a deficit between net diffusion and net phytoplankton oxygen production, and aerators have an oxygen demand. Aeration transfers more dissolved oxygen to the water in direct proportion to the amount of aeration applied. A reliable way of calculating the amount of aeration required in an aquaculture pond such as is available in aerated wastewater treatment is unavailable. Aeration rate in ponds must be adjusted by the aid of dissolved oxygen monitoring.
Diffusion also is the principle by which mechanical aerators function. Surface aerators commonly used in ponds splash water into the air to increase surface area between air and water for greater diffusion. Aerators are deoxygenators in the day when ponds typically are supersaturated with dissolved oxygen. Aeration is applied mainly at night in fish culture, but in shrimp culture, daytime aeration circulates pond water and moves oxygenated water across the bottom where shrimp usually stay.
Bottom soil is an important component of oxygen dynamics in ponds. Organic fertilizer, uneaten feed, feces and dead plankton settle to the bottom and are decomposed by bacteria creating an oxygen demand. Depending upon the intensity of culture, daily oxygen consumption by sediment may range from less than 1 mg/L to as much as 10 mg/L (water column equivalent).
A typical nighttime oxygen budget for a channel catfish pond is given in Table 2. Of course, on any given night, there may be considerable variation in the available dissolved oxygen, and the oxygen demand by all components of the oxygen budget vary among ponds. Nevertheless, the budget reveals the critical role of mechanical aeration in avoiding low dissolved oxygen concentration.
Boyd, dissolved oxygen, Table 2
Budget component | Oxygen available or required (kg/ha/night) |
---|---|
Available oxygen: Water column at dusk | 100 |
Available oxygen: Diffusion from the air | 10 |
Total available oxygen | 110 |
Oxygen demand for respiration: Fish | 60 |
Oxygen demand for respiration: Water column (plankton) | 120 |
Oxygen demand for respiration: Organisms in sediment | 42 |
Total oxygen demand for respiration | 222 |
Oxygen required from mechanical aeration | 112 |
Dissolved oxygen depletion
Several factors other than dense phytoplankton blooms and high oxygen demand can cause dissolved oxygen depletion. Extended periods of overcast skies result in less photosynthesis. Respiration is not usually effected greatly by cloudy weather, and nighttime dissolved oxygen concentrations decline more than they normally would during days with more sunshine.
Phytoplankton blooms may become senescent or they may experience sudden mortality (die-offs). Following phytoplankton die-offs there is less photosynthesis for up to a week while the bloom recovers.
Algicides, herbicides and certain other chemicals sometimes are applied to ponds. These treatments may kill enough algae that their decomposition results in low dissolved oxygen concentration.
Warmer water increases biological activity, and it also decreases the dissolved oxygen at saturation. The equilibrium (saturation) concentration for dissolved oxygen (Table 1) is 9.28 percent greater at 25 degrees-C than at 30 degrees-C in freshwater. The requirement for greater oxygen by respiration at a time when water contains less dissolved oxygen can result in dangerously low dissolved oxygen concentration.
Perspectives
Dissolved oxygen management is the most important aspect of aquaculture pond water quality management. Dissolved oxygen concentration below 3 mg/L are stressful to shrimp and most warmwater fishes and result in lower survival and production. Concentrations below 1.0-1.5 mg/L for a few hours can kill warmwater animals. Coldwater species require 2-3 mg/L more dissolved oxygen than do warmwater species.
Now that you've reached the end of the article ...
… please consider supporting GSA’s mission to advance responsible seafood practices through education, advocacy and third-party assurances. The Advocate aims to document the evolution of responsible seafood practices and share the expansive knowledge of our vast network of contributors.
By becoming a Global Seafood Alliance member, you’re ensuring that all of the pre-competitive work we do through member benefits, resources and events can continue. Individual membership costs just $50 a year.
Not a GSA member? Join us.
Author
-
Claude E. Boyd, Ph.D.
School of Fisheries, Aquaculture and Aquatic Sciences
Auburn University
Auburn, AL 36830 USA[117,100,101,46,110,114,117,98,117,97,64,49,101,99,100,121,111,98]
Related Posts
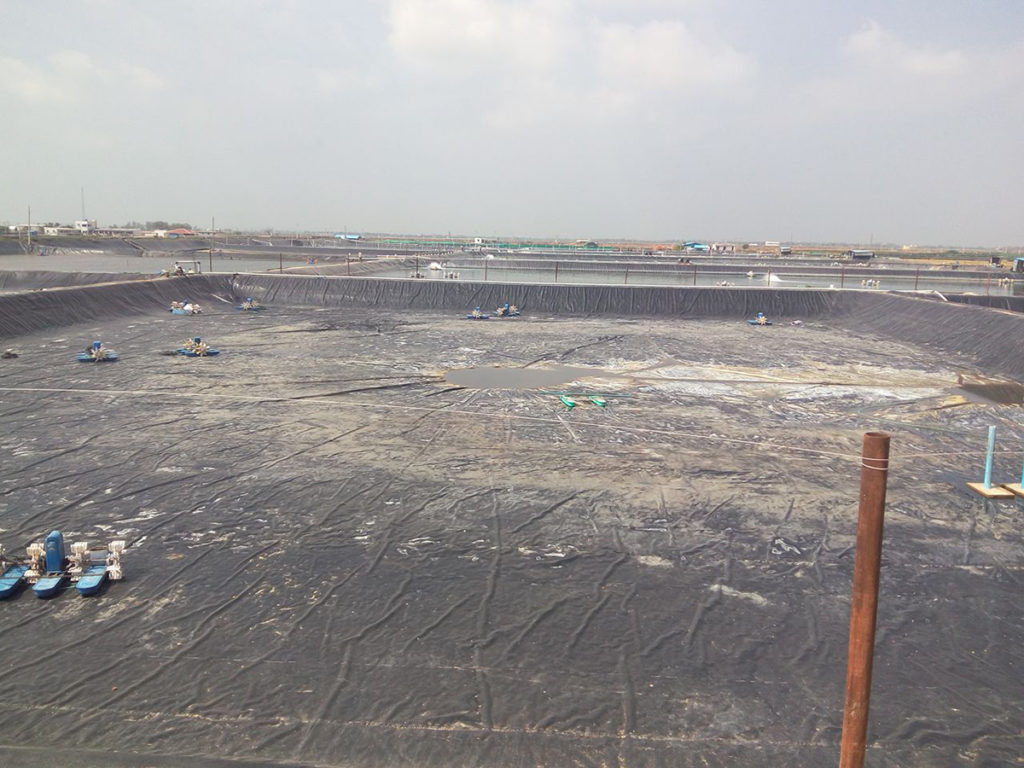
Responsibility
Appraising pond liners for shrimp culture
The use of plastic-lined ponds by shrimp farmers can significantly improve production efficiency, support more production cycles per year, and higher mechanical aeration rates and stocking densities. The capital cost of lining ponds can be very significant, so a thorough feasibility analysis is recommended when considering this production tool.
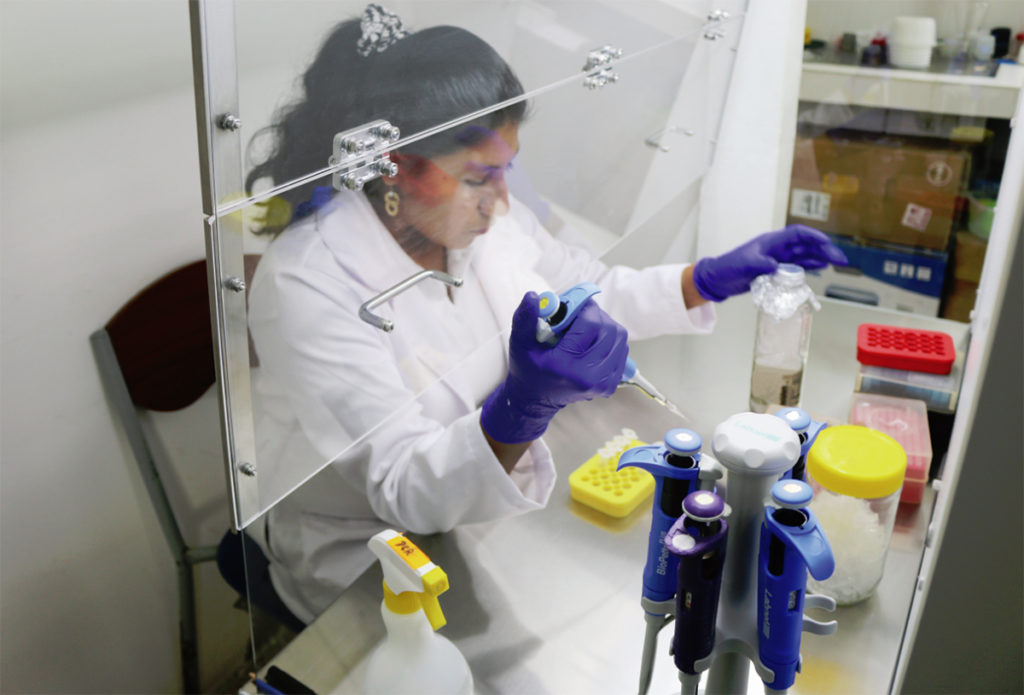
Health & Welfare
Asepsis key to prevent contamination in shrimp hatcheries
Maintaining biosecurity and asepsis in larval shrimp production is a key component of the production chain in Ecuador, which requires the production of 5.5 billion larvae monthly from 300-plus hatcheries.
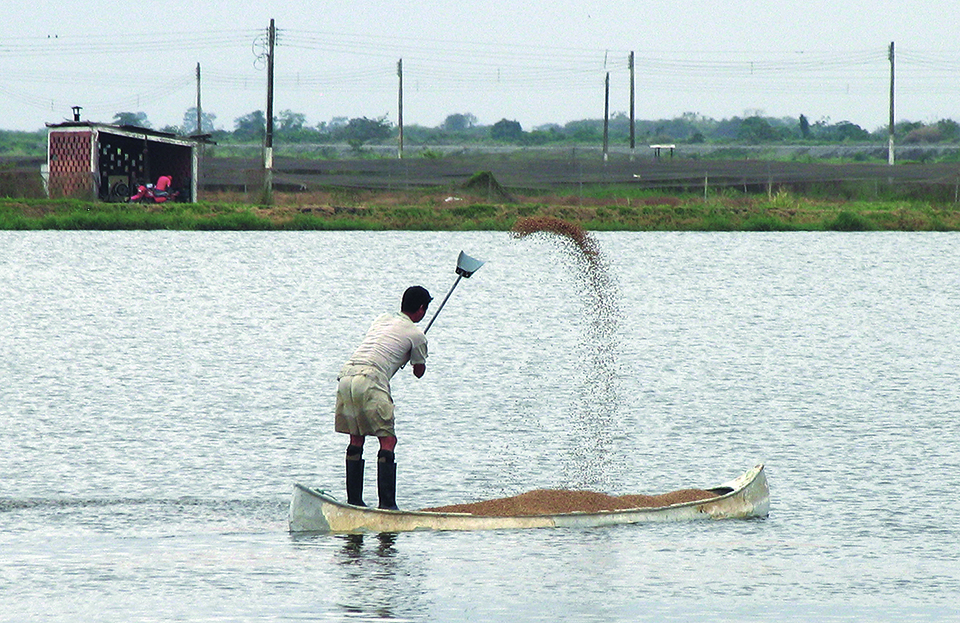
Aquafeeds
Aquaculture feed composition helps define potential for water pollution
A study found that feed for salmon and trout had higher organic carbon concentrations than did catfish, shrimp and tilapia feeds. Nitrogen and phosphorus concentrations were similar among salmon, trout and shrimp feeds, and higher than those in catfish and tilapia feeds.
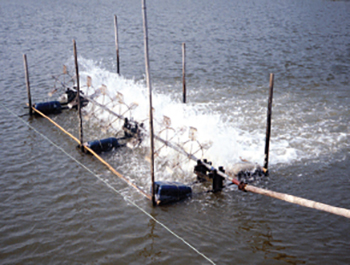
Responsibility
Efficiency of mechanical aeration
Although relatively expensive, mechanical aeration increases production. Asian-style paddlewheel aerators are widely used but are inefficient. Testing has led to more efficient designs that are now widely used in U.S. catfish and shrimp ponds.