Unicellular colonial algae grow suspended in pond water
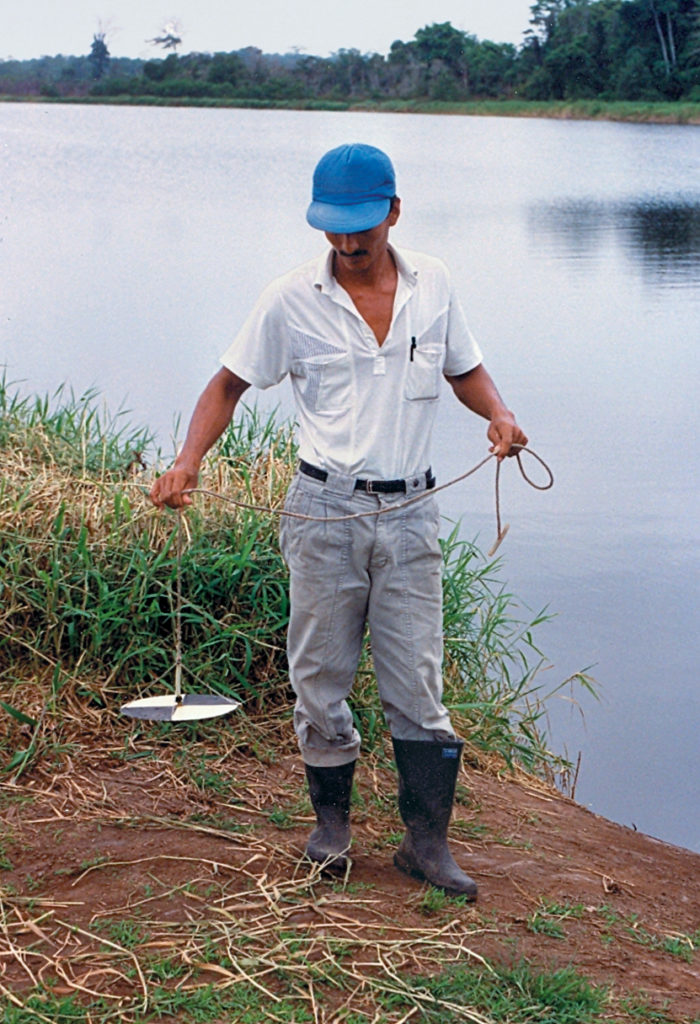
Pond managers are well aware of the importance of phytoplankton in aquaculture. These organisms are the base of natural food webs and the main source of dissolved oxygen (D.O.) in low-intensity production systems. In feed-based aquaculture, phytoplankton can become overly abundant, resulting in shallow thermal stratification and nighttime D.O. depletion.
Mechanical aeration and water circulation are employed to supplement D.O. in pond water and avoid stratification. Nevertheless, phytoplankton are essential in intensive aquaculture ponds by providing D.O. during daylight hours, contributing to the supply of natural food to supplement feed, shading the bottom to lessen aquatic weed problems, and removing potentially toxic ammonia from the water.
Phytoplankton dynamics
Phytoplankton consist of unicellular, filamentous, and colonial algae that are microscopic in size and grow suspended in pond water. They are ubiquitous in surface waters and soils, and their vegetative bodies and spores are carried by the wind. Phytoplankton do not have to be stocked in ponds, for algae quickly enter newly filled ponds from bottom soil and the atmosphere, even if the water is initially devoid of them. Phytoplankton cells require only warmth, sunlight, and inorganic nutrients for growth.
These nutrients can include carbon dioxide, nitrogen, phosphorus, sulfur, calcium, magnesium, potassium, sodium, iron, manganese, zinc, copper, and boron. In most aquaculture systems, the main nutrients that limit growth are nitrogen and phosphorus, because others are usually present in adequate concentrations.
Phytoplankton are encouraged by fertilizing extensive and semi-intensive ponds with nitrogen and phosphorus. Large amounts of these nutrients enter waters of semi-intensive and intensive ponds via uneaten feed and feces, and can result in dense phytoplankton blooms.
Photosynthesis
Phytoplankton contain light-sensitive pigments that allow them to convert carbon dioxide and water to simple sugar with the capture of solar energy in the sugar and release of molecular oxygen to the water. Phytoplankton cells use sugar from photosynthesis as an energy source in respiration. In this process, sugar is oxidized back to carbon dioxide and water with the release of energy in a biologically available form. In essence, respiration is ecologically the reverse of photosynthesis.
Sugar not used in respiration is transformed biochemically to organic compounds such as starch, cellulose, amino acids, proteins, and fats that comprise or are stored in phytoplankton cells. These compounds represent the organic matter needed by heterotrophic bacteria and animals that feed on phytoplankton cells or their remains.
Photosynthesis, respiration rates
Rates of phytoplankton photosynthesis and respiration can be estimated from changes in the D.O. concentrations in water samples. This can be illustrated by filling two clear bottles and one opaque bottle with pond water. If needed, a clear bottle can be made opaque by wrapping it with black tape and/or aluminum foil.
The D.O. concentration should be immediately measured in a clear bottle known as the initial bottle. The other clear bottle (“light”) and the opaque bottle (“dark”) are incubated in the pond for a few hours, usually from sunrise to solar noon. The bottles are then removed and their D.O. concentrations are measured.
Imagine the initial bottle contained 4 milligrams per liter D.O., and after incubation, the light bottle had 7 milligrams per liter D.O. and the dark bottle had 3 milligrams per liter D.O. In the light bottle, photosynthesis produced D.O. faster than respiration consumed it, because the D.O. concentration increased. Termed net photosynthesis, this increase can be calculated as the difference in D.O. between the light and initial bottles – in this case, 3 milligrams per liter.
No light entered the dark bottle to support photosynthesis, so the D.O. concentration fell in response to respiration. Respiration can be estimated by subtracting the D.O. concentration in the dark bottle from that in the initial bottle: 1 milligrams per liter.
The total amount of photosynthesis, or gross photosynthesis, can be estimated by adding net photosynthesis and respiration or subtracting the D.O. concentration in the dark bottle from the amount of D.O. in the light bottle. This value is 4 milligrams per liter.
For bottles incubated from sunrise until noon, the changes in D.O. concentration reflect half a daily photoperiod. During an entire day, the values would double. During the night, respiration would continue, but there would be no photosynthesis for lack of light. Respiration for a 24-hour period would be 4 milligrams per liter in the example. The 24-hour value for net photosynthesis must be reduced by 2 milligrams per liter to account for nighttime respiration. The net photosynthesis value of 4 milligrams per liter represents the D.O. available for nonplanktonic, aerobic organisms in the pond.
Light and photosynthesis
The influence of light on photosynthesis causes the well-known daily fluctuations in D.O. in ponds, with concentrations lowest at sunrise, a rise to a peak in the afternoon, and a decline during late afternoon and night. Increases in phytoplankton abundance result in greater afternoon D.O. concentrations and lower nighttime concentrations. Decreases in phytoplankton have the reverse effect.
When skies are clear, greater solar radiation favors higher D.O. levels, while cloudy skies favor lower concentrations. Unless water temperature declines, respiration is not affected by cloud cover. The sudden introduction of turbidity from suspended soil particles after rainfall or for other reasons reduces light availability and has the same effect on D.O. concentrations as cloudy weather.
Natural phytoplankton die-offs or the introduction of agents toxic to phytoplankton will lessen photosynthesis, but often increase respiration due to bacterial decomposition of dead phytoplankton. This is why algicides such as copper sulfate cause a decline in D.O. concentration.
Pond depth and photosynthesis
Photosynthesis decreases with depth in ponds because light availability declines with depth. The depth at which net photosynthesis and respiration are equal is the compensation point (Fig. 1).
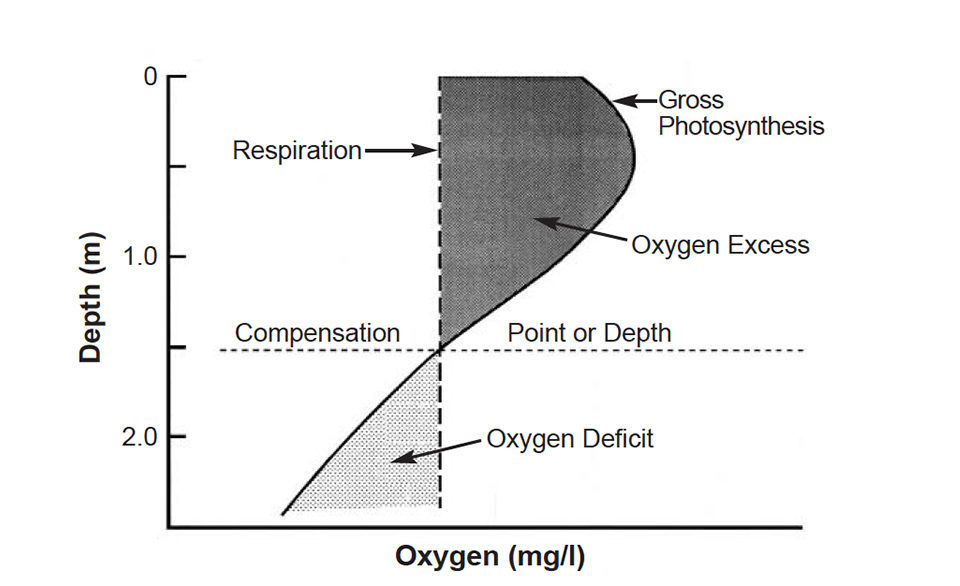
Since aquaculture ponds are shallow, their waters mix completely at night. If excess D.O. in the water column above the depth of the compensation point is greater than the deficit of D.O. below this depth, more D.O. is produced in the water column than used. The opposite occurs when the D.O. deficit below the compensation point is greater than the excess above it.
If a pond produces more D.O. than that used by respiration in the water column, the difference is available for sediment respiration and respiration by the culture species. Of course, during the night, when D.O. concentrations are below saturation, oxygen enters the pond water by diffusion from the air.
The oxygen that enters by diffusion seldom exceeds 1 milligrams per liter during a single night. Nevertheless, diffusion can be very important in preventing D.O. depletion at night. Mechanical aeration must be applied or increased where the amount of D.O. produced in the water column plus that entering by diffusion is less than the amount of D.O. needed by sediment and the culture species.
Ponds should have a compensation point at which surface light is prevented from penetrating to the bottom to cause aquatic weed growth. The depth of the compensation point can normally be taken as twice the Secchi disk visibility. Assuming that most managers maintain a Secchi disk visibility of 30-40 cm, minimum pond depth should be about 100 cm to avoid underwater weed problems.
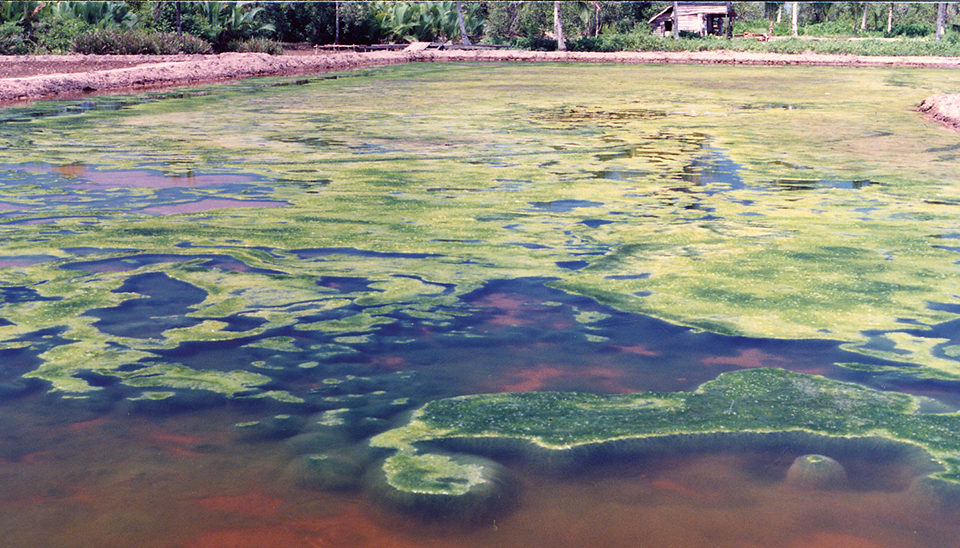
Shrimp production
Some shrimp producers have increased stocking and feeding rates to such high levels that the water becomes so turbid phytoplankton are greatly limited by light. Dissolved oxygen is maintained in such ponds by intensive mechanical aeration, and the water column becomes dominated by bacteria rather than phytoplankton.
Such systems can be quite stable with regards to water quality because dissolved oxygen is maintained by the aerators and nitrifying bacteria prevent ammonia from accumulating to toxic concentrations. The bacteria in such systems degrade organic wastes from feeding and also serve as supplemental food for shrimp.
(Editor’s Note: This article was originally published in the November/December 2006 print edition of the Global Aquaculture Advocate.)
Now that you've reached the end of the article ...
… please consider supporting GSA’s mission to advance responsible seafood practices through education, advocacy and third-party assurances. The Advocate aims to document the evolution of responsible seafood practices and share the expansive knowledge of our vast network of contributors.
By becoming a Global Seafood Alliance member, you’re ensuring that all of the pre-competitive work we do through member benefits, resources and events can continue. Individual membership costs just $50 a year.
Not a GSA member? Join us.
Author
-
Claude E. Boyd, Ph.D.
Department of Fisheries and Allied Aquacultures
Auburn University
Alabama 36849 USA[117,100,101,46,110,114,117,98,117,97,64,49,101,99,100,121,111,98]
Tagged With
Related Posts
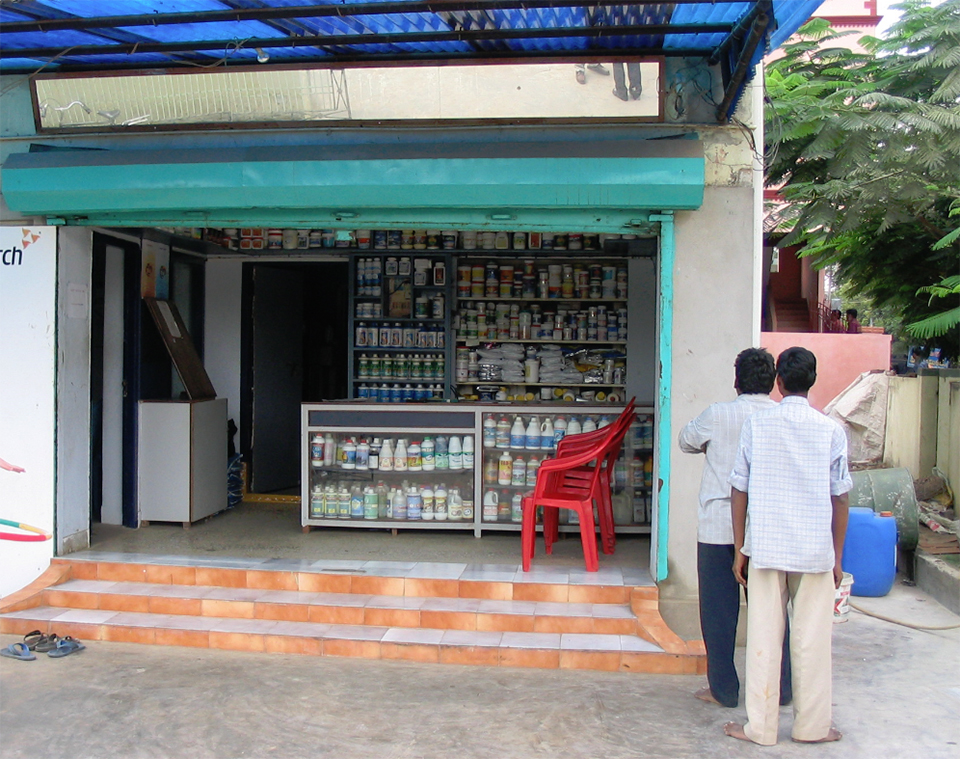
Responsibility
A review of water quality improvement products
Prof. Boyd examines products used by aquafarmers to improve water quality and conditions in their ponds and discusses their efficacy.

Health & Welfare
Add value to shrimp harvests: Darken environment to enhance shrimp color
Shrimp color is caused by pigments that play an important role in camouflage for survival. For farmed shrimp, proper coloration is generally achieved via synthetic astaxanthin in feed.
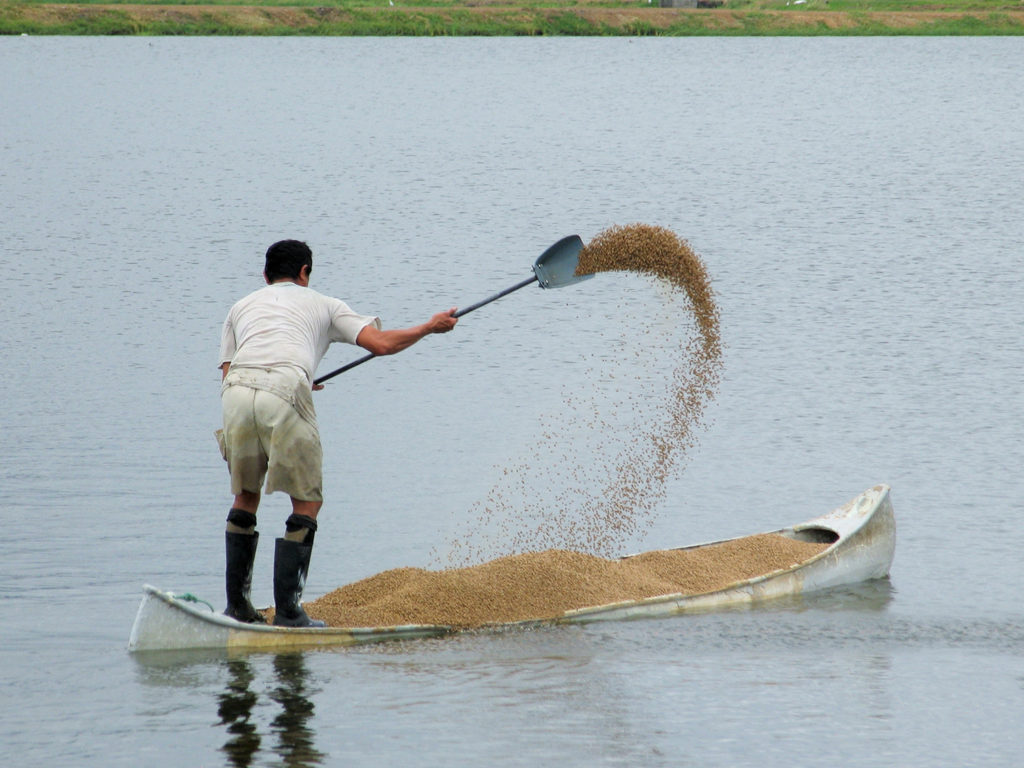
Responsibility
Ammonia nitrogen dynamics in aquaculture
The major sources of ammonia in aquaculture ponds are fertilizers and feeds, and problems with high ammonia are most common in feed-based aquaculture.
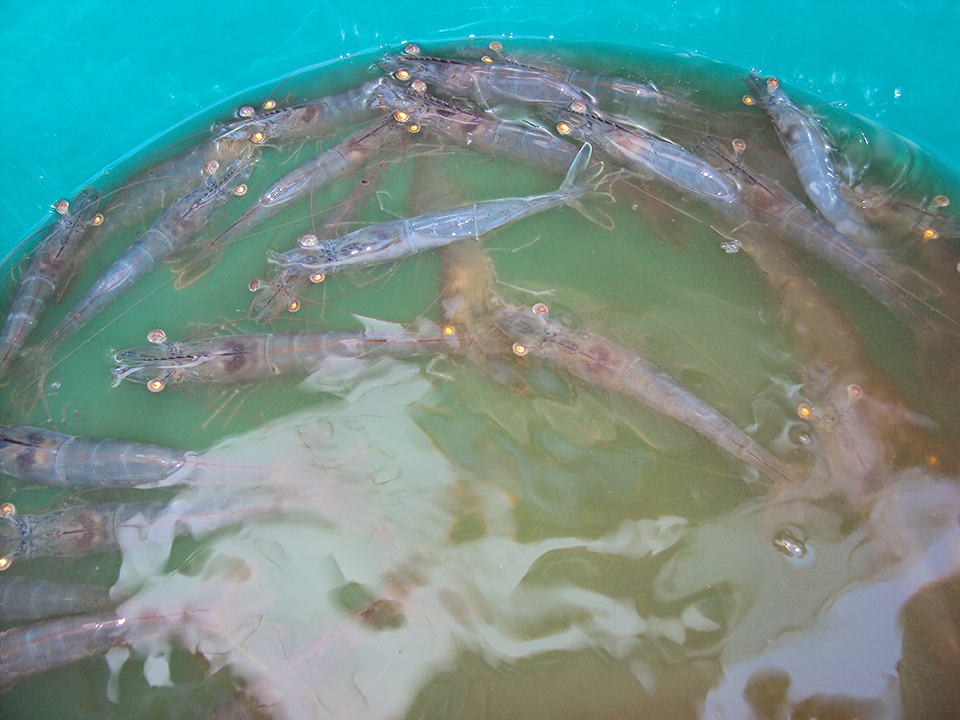
Health & Welfare
Ammonia toxicity degrades animal health, growth
Ammonia nitrogen occurs in aquaculture systems as a waste product of protein metabolism by aquatic animals and degradation of organic matter, or in nitrogen fertilizers. Exposure can reduce growth and increase susceptibility to diseases in aquatic species.